Flash and JavaScript are required for this feature.
Download the video from iTunes U or the Internet Archive.
Topics covered: Space Shuttle History
Instructor: Prof. Cohen
Subtitles are provided through the generous support of Heather Wood.
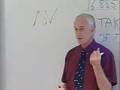
Lecture 2: Space Shuttle Hi...
The formal part of the class will start about five past nine, but I'll just start with some introductory remarks.
First of all, I have got bios from a large number of people.
I haven't done a count yet to see from whom I haven't gotten them, but if you haven't sent me a short bio please do.
It just helps us to get to know who you are.
Let's see.
Second thing.
The next deliverable, which is, I think, in a week or so, I don't have the syllabus with me right now, we would like a preliminary indication from you.
I am leaving it up to you to form your own teams.
Roughly, we've found about 4 people make up a good team.
That is a good way to spread the work around.
If any of you are having difficulty or whatever, let me know, we will try to help, but you are almost all graduate students.
You can organize yourself.
With a preliminary indication of what system you would like to do a study on.
Take a handout.
I am just wondering if you can provide a list of email addresses to everyone so that we can contact each other.
I will be happy to do that.
I will post that.
Let's see.
The second thing.
There are a fair number of listeners in the class, and quite a few of you have contacted me to make sure that it is OK to be here as listeners.
I have no problem with that.
The only thing that I would ask, right now we are more or less filled to capacity.
I expect that the class isn't going to grow after this, so I think we are OK.
What I did tell people is that if we get into a seat crunch, obviously, the people who are taking the course for credit have priorities for seats.
So any of you who are listeners please keep that in mind.
Next thing.
I am going to be putting some things on reserve in the library.
This is courtesy of Professor Cohen.
This is the proceedings of a Space Shuttle Technical Conference.
Aaron, do you want to say something just about the background?
The background is that we decided, after the Shuttle had flown a couple of times, that we would have a typical NACA, going back to NACA, but this was NASA technical conference where we actually had technical papers written by the various people that designed the system, some you will hear talk, some you won't.
But these are technical papers of how the systems were developed.
So it is a pretty detailed understanding of the thermal protection system, the main propulsion system, so forth and so on.
So it is a pretty good documentary of how it was done.
This should be a good reference for your papers.
It is 1983.
Like I say, I will go down this afternoon and put this on reserve in the library.
I will also put on reserve a copy of a systems study that was done at Texas A&M for a similar course that Professor taught on systems engineering and the Space Shuttle just to give you kind of an idea of what someone else did.
This was a rather good paper.
I see you gave them a very high mark so presumably that means it is a good example.
Finally, you have all got a copy of this paper by Professor Cohen and Milt Silvera who was also a very responsible person in the Shuttle program.
It is a high level background on the Shuttle and its systems.
I am sure there are some of you here who are space enthusiasts and who probably have followed the Shuttle system pretty closely and to whom there won't be a whole lot new here.
But, on the other hand, we want everybody in the course to come up to a certain level of knowledge about the Shuttle, its systems and the systems engineering that went behind it.
So I think it's good to have something like this available.
It's a nice reference piece.
Today and Thursday Professor Cohen is going to give an introduction into the Shuttle, its systems and the system engineering that went into it.
Before we start that, are there any other questions either about the technicalities of the course or just anything?
Oh, I know one thing.
I have a few comments from people telling me that the pdf files that I posted on the website, some people could read them and some people could not read them.
I have Macs but have been posting pdf files for numerous courses.
I have never had any problem with people reading it before.
First of all, who has tried to look at the files?
OK. Of those people who have tried to look at the files, who has had difficulty?
This is strange.
Well, if anybody has any ideas about what the problem might be, I would be very curious.
I mean have you ever had this problem with other courses?
I will do a couple of experiments and post one or two more documents, both in pdf and in either Word or PowerPoint form.
I would suggest that everybody during the next two days go and try to open them.
Let's see if we can isolate where the problem might be.
Like I said, I've never had this problem with any other courses.
And, in principle, I mean the whole point of pdf files is it's supposed to be compatible across Macs, PCs and all that.
So I don't know what the problem is but we'll see if we can run it down.
And thanks to those of you who let me know that there was a difficulty.
That is all I have.
Any other questions, comments?
What are you kind of expecting with these journal deliverables so we can kind of be just ahead of that?
The idea is to basically put together the highlights of the lecture from the systems engineering point of view.
In other words, what we want -- When somebody is talking about a specific system, we want you to have a brief description of the system, the purpose, what it does, something about the design considerations, something about the operations, kind of the basic concerns from the systems engineering point of view.
And anything they talk about, particular interactions between that system and other systems.
Can you think of anything else in particular?
I think that about it.
OK.
I will think that through a little bit more, and maybe I can come up with a checklist that would help you put that together.
It's not meant to be a big burden.
It's just a way to organize everybody's thinking on how to get the most out of the lecturers, particularly because, as I mentioned, these lectures are all being given, by and large, by different people.
They will have different styles.
We have kind of explained to everybody the structure of the course, what we're looking for, but I cannot really guarantee in advance what the content of the lecture will be.
Professor Cohen and I will make any attempt, if the lectures don't cover some of those critical points from a systems engineering point of view, we will try to stimulate the discussion on that or fill in.
But you should do the same thing.
In other words, make sure that there is something that you think you would like to be getting out of the lecture and you haven't gotten, don't be embarrassed to ask questions.
Something you ought to think about, when these individual lectures come up, is it should be the description and function of the system.
If they don't bring that out then we ought to try to bring it out.
The requirements of the subsystem or the system, what are the requirements?
Because that is very fundamental.
What are the requirements?
The development of the subsystem.
What kind of problems did they have?
What kind of technologies did they have to overcome?
And, of course, the operation of the subsystems.
Those are, I think, key points.
Now, whether you're going to get that from everybody or not, I don't know.
But I think you need to look for that.
And, if you don't, then we will try to develop that.
Are you looking for the notes to be taken in class?
I don't follow you.
I mean I am not looking for a Xerox of what you're writing in the notebook, if that's what you mean.
No.
I mean I would like you to put your thoughts together into a concise form.
And I think that will be a good reference to you, hopefully, after the course on Shuttle systems.
OK?
Over to you.
You are going to have a very unique opportunity.
You are going to have people speak, lecture you on the various Shuttle subsystems in quite a bit of detail.
You will have some people that will be very, very positive about the Shuttle, that think it's a great design, a great operation.
You will have others that will not think it's so good.
And you will have some that will give you just a detailed technical approach of what happened.
What I would like to suggest to you, I think, for your good and for the good of the future, future students, future designers, is that you ought to come up with your own decision.
Was it the right design?
Was it the correct design?
Should it have been done differently?
And, if so, why and how would you do it?
And I think it will be good for, when you go to work on future projects.
And it would also be good, I think, for NASA, something we could turn over to NASA.
I think it's a very valuable thing to do.
So I would suggest you do that as you go through the course.
And I will be very happy to talk to you about any of your ideas that you have through the Internet, through emails or in person discussion.
And you might want to do this later on as the semester develops.
A little bit what I am going to say today really starts off where Dale Myers, your previous speaker, left off.
And it gets into a little bit more detail.
So, as this course develops, you're going to get more and more detail.
But let me start off again, just very much like Dale did, and talk about the Shuttle history.
In 1952, the fully reusable launch vehicles concept was discussed.
People were interested in that.
1962, fully reusable vehicles were seriously considered.
The Air Force study project Dynosaur was cancelled in 1969.
In 1969, NASA adopted the idea of a fully reusable spaceship.
I became the Orbiter Project Manager for NASA in August of 1972.
And, at that time, I also was manager of the Systems Engineering Organization for the first two years.
So I, you might say, the total system at that time and the Orbiter in 1972.
Yes?
Could you tell us a little bit about your background?
Yes.
Well, my background was I graduated from Texas A&M University in 1952 and went to the Army, went to Korea.
And then when I came back went to work for RCA.
And I worked on the microwave tubes, the microwave oven.
In fact, when I told my wife what I was working on, I said I'm working on a microwave oven.
That was in 1954.
That was a long time ago.
How many people have microwave ovens today?
Everybody does.
Well, in 1954-1955, when they came out, they were about $3,000 a piece.
I was working on one and told my wife.
And we'd been married a long time.
I told my wife that I was working on something called a microwave oven.
And you are going to be able to cook a roast in a couple of minutes and a potato in a couple of minutes.
And she looked at me and said that will never sell.
Anyway, that's what I worked on.
Then I worked for General Dynamics on the Atlas and Centaur.
And then, in 1962, I went to the Johnson Space Center and worked very closely on the Apollo Program, worked very closely with the MIT Instrumentation Lab, now the Draper Lab on the guidance and navigation control system.
I became head of System Engineering in Apollo.
Then manager of the Command and Service Module in Apollo.
Then, in August 1972, I became the manager of the Space Shuttle Orbiter.
Then I became Director of Research and Engineering at Johnson Space Center.
And then I became Director of the Johnson Space Center.
And then, for a while, I was the Acting Deputy Administrator in Washington.
And then I retired and went to Texas A&M to teach.
Then Jeff asked me to come to do this, and I am very happy to be here.
Actually, one thing you mentioned reminded me of something, not to divert the lecture today, but the fact that there was specifically a systems engineering group at the center which was separate from the project offices is something which is probably worth talking about at some point.
That's right.
Well, let me just make a mention of that.
When we were in Apollo we sat around the table for many days and months trying to figure out how you define systems engineering.
We didn't even know what systems engineering was.
In fact, today, I'm not sure you'll get a clear definition of what it is.
I want to give you some examples.
As we go through the lecture, I am going to give you some examples of what I think it is and how it was used.
So we will do that.
Let me say just one other thing.
Professor Cohen has these view graphs in electronic form down in Texas and, when he gets back, he will send them to me and I will post them on the website.
Well, I will give you this whole package because there are also some pictures.
I don't know what you want to do with the photos.
Something that is very key in any design and something you really need to pursue, whenever you do a design project, is understand the requirements.
Because, if you don't understand the requirements, you might get a very good product that is useless.
So you have to understand what your customer wants, the top level requirements.
One thing that was handed down to us is it was supposed to be fully reusable.
That was one requirement.
14-day turnaround time.
You were supposed to be able to turn the Shuttle around in 14 days.
Deploy and retrieve payloads.
You had to deploy a payload and you had to retrieve a payload.
Design, development and test is estimated to be $5.1 billion in 1971 dollars.
Dale Myers didn't tell you the whole story, but one reason Dale Myers and I are such good friends is he was Associate Administrator of Manned Space Flight, I was the Orbiter Project Manager and they, they being headquarters, headquarters was always there to help, actually took away two years of inflation.
If they had given those two years of inflation, we would have met the $5.1 billion in 1971 dollars.
And Dale fought for that but lost.
Now, here is where we missed it.
The original cost per flight for 65,000 pounds was $10.5 million per flight in 1971 dollars but for a flight rate of 60 flights per year.
When I told my wife I was doing that, and she's been around the space program a long time, she said you never agreed to that, did you?
Sixty flights per year is pretty hard to do, but that's what we came up with at the time.
Now, Dale mentioned the Air Force requirements, but here were the phase A studies.
The phase A studies were conducted to determine the basic requirements and their effects on design in 1969.
The principle issues were the size and weight of the payload, the cross-range of the Orbiter and what kind of heat material was going to be used.
You have to recognize are we going to use heat-resistant structure or reusable insulating material?
You have to recognize that our background was Mercury, Gemini and Apollo, and they all used an ablative material.
Ablative material cannot be reused because basically the surface changes.
You had to have some kind of insulated material so the surface did not change.
Let me now go through very quickly for you, just for the sake of completeness, Dale showed you a few of the studies.
I want to flash up a lot of phase A studies just to let you take a look at what people were doing.
And you don't have to read the words but you might just look at the diagram.
There was General Dynamic's phase A study.
There was North American Rockwell.
That is the one Dale was talking about because that was where he was.
And there was a phase B study for McDonnell Douglas.
So that is what they all looked like at the time.
Now, I am going to go through some just for the sake of completeness here.
There was, again, another McDonnell Douglas study, Martin Marietta.
So those were basically some of the studies.
You can see they all had some kind of a wing-type vehicle.
Already most of those were delta wings so the decision had already been made.
Interestingly enough, and I am going to quote some names to you, and you might go back and research it.
Max Faget was like the lead engineer.
He was sort of a man that was immortalized in terms of the space program.
He felt we ought to go with the straight wing.
He felt very strongly about a straight wing, but that eliminated the very large cost range the Air Force needed.
Straight wing was easier to build, not as high loads on it and so forth.
Here, interestingly enough, if you look at Chrysler.
Chrysler had a study.
And Chrysler actually had a capsule, but here were more vehicles.
And people were really thinking about this time of a fly back booster where you actually had a booster that returned and came back and landed.
And they also had a land vehicle that landed.
So that was what the real requirement was at the time.
Somebody sort of commented about Chrysler.
There was also Ford.
There were a lot of aerospace companies back in those days.
It has changed a lot, that's right.
And here is McDonnell Douglas, of course Grumman.
So a lot of these studies.
Then you're getting to see something already starting to look a little bit like the Shuttle.
And I am almost through.
And here are more.
These are, I think, getting maybe in the phase B studies.
But you can see some of them are starting to look like the existing Shuttle.
Lockheed.
Now, the principle issues in the Shuttle studies, now we're starting to get a little bit more technical and a little more detailed, is should the reaction control system, now, the reaction control system is basically a propulsion system that controls the vehicle about its center of gravity.
It's for alttitude control, basically.
Should the reaction control system be liquid oxygen/liquid hydrogen or should you use a hypergolic system where you know it is a storable type system.
That was a big issue.
Does everybody here know what hypergolic fuel is?
Let's talk about it a little bit.
Well, hypergolic fuel is a fuel like hydrazine.
It actually has an oxidizer and a propellant in the same fuel.
Now, another issue was a fly-by-wire flight control system.
That was a big issue.
Do we have a fly-by-wire flight control system?
And now everything has a fly-by-wire.
All the military jets have fly-by-wire.
But basically was it going to be a computer controlled system or are we going to have cables fly the machine?
That was a very big issue at the time.
Wind tunnel tests to determine wing size and configuration.
That is a very difficult thing to do.
This is starting to get into what you might say is systems engineering.
Air breathing engines were considered for fly back and later determined to be too heavy.
And some Shuttle studies that still had to be done was the entry techniques, landing speed, what type of landing speed were we going to have and the approach pattern?
So these were all some things that had to be understood during the studies.
The phase B studies were performed in the mid 1970s to determine the preliminary design.
The results showed a fully recoverable Orbiter.
Disposable fuel tank.
Parachute recoverable solid rocket boosters.
High performance hydrogen oxygen engines placed in the Orbiter to recover.
This was all systems engineering that lead up to the design.
So you have systems engineering in various phases of the program.
And usually systems engineering composes of an interdisciplinary team that has been given some assumptions, some constraints.
They have some top level requirements.
They do an iterative process with some tools such as computer tools for calculation of loads and flight mechanics.
And they come up with an iteration of what the design is going to be.
So that is basically how it was done.
Now, some of that was sort of like the ground rules.
Some of the things that came out of it, once we started putting the total system together, we showed that the fully reusable with a fly back booster was greater than $5.1 billion.
So that was thrown out.
Now, there is a question, and that's what I asked Dale, should we have said, hey, we need to money to really have a fly back booster?
But they gave us a constraint of $5.1 billion in 1971 dollars and it didn't make it.
I showed you, many configurations were studies.
And the turnaround time of 14 days dictated a landing with a winged vehicle on a runway.
You weren't going to be able to land this in the water or with parachutes.
You wouldn't have to land it on a runway so you could quickly turn it around in 14 days.
The payload, deployment and retrieval requirement determined the location of the Orbiter and the launch configuration.
If you look at that large payload bay, it would be very difficult to put that on top of the vehicle.
One of the requirements that NASA has now is the CEV should be on top of the stack.
Well, if you're going to have that large a vehicle, it is pretty hard to put it on top of the stack, particularly with wings.
This is what we had to come up.
These are some of the results.
And these are all systems studies.
This is systems engineering.
Now you're getting to look at what the design is starting to look like.
This is the agency commitment in March of 1972.
In May of 1972, you had the North American Proposal.
And then I became Orbiter Project Manager in August of 1972.
We did a study.
And PRR is the preliminary requirements review.
But that was the configuration.
We made some changes.
And the production commitment was made in May of 1973.
This chart, which was sort of the gee-whiz chart at one point in time, showed the 1971 dollars cost per flight for the Thor, the Atlas, the Titan 3c, the Saturn 1b and the
Shuttle. And that is payload to orbit.
So you can see that the thing that was really missed in the Shuttle was the $10.5 million cost per flight.
Yes, sir.
What is the notch on top of the second two that disappeared?
That up there?
That was their data probe.
It was taken off because it wasn't needed.
Good question.
Thank you.
And, by the way, do not hesitate to interrupt me and ask me questions any time.
And, if you don't understand what I'm saying, please stop me, tell me slow down or ask me any questions you would like to ask me.
There are data probes on the Shuttle.
Major configuration decisions.
And, by the way, you don't see them on the Shuttle because they are inside the thermal protection system.
And they are only sort of turned outwards to take data when you get down to about mach 3 and you're through the heating.
Otherwise, they would burn off.
In the approach and landing test they were out front.
When we separated from the 747, I am going to tell you that story in a minute.
Here are some of the major decisions.
We were going to go with a hydrogen/oxygen main engine.
That is one of the system problems you have to decide.
Once you decide what kind of engine you are going to use, that basically sizes the tankage.
And I will show you that on another chart.
As I said, this size, the liquid oxygen/hydrogen tanks are not reusable, and I will make that point to you a little later on, but once you decide what kind of engine you are going to use, that size of the tank because of using the equations of motion you can figure out how much propellants you need, you get the density of the propellant and now you know what size tank you are going to use.
Yes, sir.
A couple slides back you had said the main engines of the Orbiter had to be recovered.
Right.
[AUDIENCE QUESTION] It was a separate contract, but it was placed inside the Orbiter.
When the Orbiter came back the engines came back with it.
Was that your question?
Yes.
For example, the Johnson Space Center was responsible for the Orbiter.
And you're going to have the person talk that was responsible for the engine, J.R. Thompson.
He was at the Marshall Space Flight Center.
But the engines were installed in the Orbiter so the Orbiter brought them back.
Solid rocket boosters provided the additional propulsion required to get the Orbiter in orbit and the solid rocket boosters were designed to be recoverable and reused.
Those were some of the system studies that led to the configuration.
Yes, sir.
At that period, was there any discussion of the environmental impact of solids being used at 60 flights a year?
Yes there was.
There was quite a bit.
I don't recall the details, but I do know there was a lot of work on that many solids being used.
And I guess we basically put that to rest, but there was a lot.
I don't know the details of it.
In fact, that might be a good question.
If you are not here, we will ask J.R.
Thompson because J.R.
should know that.
I met with some of the Russians who worked on the Buran which was the Russian comparable to the Shuttle.
One of the big changes was that they said how could the Americans have used solids for that many flights?
It was studied and actually put to bed, or put to rest, should I say?
I don't know the details, but that is a very good question.
There are going to be various speakers, as I said, and J.R.
should have that answer.
Solid rockets recovered and reused.
Well, some of the things I have said before, the Orbiter entry cross-range required delta wings.
To go 1100 nautical miles cross-range you needed delta wings.
Deletion of the air breathing engines for moving the Orbiter required the Boeing 747 to carry the Orbiter.
Let me tell you that story.
All of you are very familiar now that when we land at Edwards Air Force Space, we put the Orbiter on top of the 747 and we fly it back to Kennedy.
Well, I was the Orbiter Project Manager, I became Orbiter Project Manager August of 1972, and I was having all sorts of problems.
The first thing they did to me was cut my budget in half.
The OMB cut my budget in half.
That was the first thing that happened.
And then I just had a lot of problems.
But I had worked on the Apollo Program.
I had a lot of friends in the organization, although I was Orbiter Project Manager.
Three of my friends came into the office one afternoon, I forget, maybe two or three months after we started, and said Aaron, we have a great idea.
I said what's that?
They said we can put the Orbiter on top of a 747 or a DC10 and ferry it back to Kennedy from Edwards Air Force Base.
We may have to make one or two stops and ferry it back.
I look at him for a moment and said that is absolutely the dumbest idea I heard in my life, and I basically threw the people out of my office.
And these were my friends.
Well, these people will not take no for answer.
It happened to be they had another very good quality.
They were all world-class model airplane builders.
And these guys had won competition all over the world, three of them.
So they came back about ten days later.
And I don't know how many of you have seen the Johnson Space Center but we have a lot of acreage out there in Texas.
They said come out, we want to show you something.
They had built a radio controlled model of the 747 and an Orbiter and actually flew it for me and separated the Orbiter from the 747.
That is how it got started.
And so we eliminated the air breathing engines.
But I remember throwing them out of the office.
Fly-by-wire with a digital autopilot.
Yes, sir.
Are you saying that you had air breathing engines and the Orbiter itself would fly back to Edwards Air Force Base?
That's a good question.
I missed that point.
Let me explain it to you.
That is exactly right.
If you recall, when the Orbiter lands, the nose gear is very short.
What we had to do, he asked a very pertinent question, we had to actually replace the landing gear with a different landing gear that caused the Orbiter to have an attitude like this.
It wasn't like this.
It was like this.
We put air breathing engines on and took off and had to have five in-flight refuelings to get to California.
Strap on air breathing engines.
And, plus, a different landing gear.
And we took off horizontally.
And five in-flight refuelings to get from California to Florida.
And you brought up an important point that I left out, that's the reason why we changed it.
Thank you very much.
And, of course, we went with the fly-by-wire with a digital autopilot.
This was a very fundamental change.
The astronauts at that time did not like this very much.
Now, when you get all these new pilots in, they wouldn't know what you were talking about.
Why not have a fly-by-wire system?
They didn't like it at one time, but we went with a fly-by-wire with a digital autopilot.
Even though you are going to have a special briefing on the guidance navigation control system, I am going to talk a little bit more in detail about that because that happens to be my expertise.
So I am going to talk about that a little bit in more detail.
Yes, sir.
What does fly-by-wire mean?
It means that you actually have a computer which actually controls the surfaces.
Whereas, in past airplanes, your stick actually had cables that controlled the surfaces.
So you get a lot more performance.
It is a little bit confusing in the sense of a wire.
Don't think of it as a hard wire which is like the old type of airplanes where there was a cable.
When you pulled on the stick there was actually a cable that went back to the ailerons and the rudder and everything.
And, as professor Cohen says, everything now has a computer in the middle.
And what you're really doing is flying the computer.
And the computer then issues the commands to the hydraulic system.
But the Shuttle, I guess, was the first vehicle that really had that system.
There were no commercial planes flying with that system or military planes flying.
And the real concern was safety and reliability.
Suppose you have a computer problem, what are you going to do?
I will talk about that in detail.
That's a good question.
Any other questions.
Those are very good questions.
I appreciate them.
Yes, sir.
Can you just explain cross-range for a moment?
Cross-range.
Realize the Orbiter is a glider so-to-speak.
Not much of a glider but a glider.
And, actually, downrange would be going in this direction.
Cross-range would be out of plane direction, so you could actually maneuver out of plane.
We have a nice globe here.
I am not going to carry it up to the front and I don't have a piece of chalk either.
These are very good questions, by the way.
Thank you very much.
Here is the United State roughly.
This was designed for the military requirements.
They wanted to be able to, for reconnaissance satellites, basically, you want to be in polar orbit because you are going around, let's say this is an orbit here, then the earth turns underneath it, and so you basically fly over all parts of the earth.
That was the basic military requirement.
They wanted to be able to launch out of Vandenberg on the West Coast into a polar orbit.
And, because of security reasons, this sounds a little bit strange when we think back on it, but in a time of crisis, remember we were in the Cold War and everything, you wanted to be able to put a satellite up without necessarily giving the other side a chance to make all the radar measurements on the Shuttle and everything and figure out right away where the satellite is.
And also there might be hostilities.
The Shuttle was a strategic asset.
So basically they wanted the Shuttle to be able to land the very next orbit.
Well, all right, you take off from California, you fly over the pole and you deploy your satellite.
And, by the way, we have never, with all the satellites we have deployed, ever deployed a satellite on the first orbit.
That would be an incredible feat, but that was the requirement.
So you fly over the pole, you come back around.
Now you're ready to land in California.
But, during that time, the earth has turned by a thousand miles, 24,000 mile circumference in 24 hours.
Actually, 1500 miles because an orbit is 90 minutes, 1.5 hours.
Your orbit now would put you right over the Pacific Ocean.
If you just burn your engines, slow down and come down through the atmosphere, that is where you are going to land.
So instead, as you're flying through the atmosphere, you basically have to come down banked on your side.
So, essentially, you're generating a lift vector.
And, instead of turning your lift vector up, you turn your lift vector to the side and that pushes you over.
And delta wings can generate a higher lift vector than the straight wings, and that was the determining factor.
Very good.
[AUDIENCE QUESTION] Well, I think that's right.
Can you address that, Jeff?
My recollection at the time was that even coming in over the Pacific, yes, there were some places where you could land on land but it would mean landing in hostile territory, the Soviet Union or Eastern Europe, and we didn't want to do that.
So the cross-range initially was to be able to make Australia.
Well, I'm sure that's right.
Certainly, there were landing sites that we had all over the world.
It could have been a much smaller cross-range if we were willing [OVERLAPPING VOICES].
And, I will say, continue to remind us if we don't explain some basic things.
Because we've been dealing with this for so long that some of these things just seem like second-nature.
You weren't even born when the Shuttle started flying.
And so the level of backgrounds is going to be very different.
We want to bring everybody along with us.
So if there is something that we say the same thing, you know, the question about hypergolic fuel, if we use a term and you don't understand it, we will try not to use a lot of acronyms.
I cannot guaranty that the speakers won't use acronyms.
Don't be embarrassed to just stop and say what does that mean, what are you talking about?
But, as we go through the course, you are going to get more detail, more detail.
For example, you have a total lecture by a man named Henry Pole who is going to talk about the reaction control system.
He will give you all the details you want to know about hypergolics and storables, more than you ever wanted to know.
So you are going to get more details as you go through.
But don't hesitate to ask questions because it's good for us.
Let me tell you another satellite story.
My wife and I moved to College Station.
My wife was working at a junior college in the registrar's office.
She was working with a young lady and Apollo 13 came out.
Well, Apollo 13 happened to be my first mission when I was Manager of Command and Service Module so I know a little bit about it.
In fact, I am going to give a lecture in Dick Batten's course on the 26th on Apollo 13.
But my wife talked to this young lady and said I went to see Apollo 13 yesterday.
And she said it was so exciting.
I didn't know how it was going to end.
[LAUGHTER] So it is a frame of reference.
Well, we've talked about this.
The size of the payload bay is 60 feet long by 15 feet diameter.
Size of the crew cabin defined to be over 2600 cubic feet.
The payload, as you know, is 65,000 pounds at liftoff, 35 pounds at landing.
And what you need to understand is the Orbiter is a launch vehicle, it's a spacecraft and it's an aircraft.
And when you look at the two different systems, I worked on Apollo, as I said, very much and on the Shuttle, and there is no question that the Shuttle is much more complicated than Apollo.
On the other hand, the Apollo mission is much more complicated than the Shuttle mission.
But this is something that makes it very, very interesting.
This is a very old chart.
And, in fact, this is the original chart.
It comes out yellow.
This was the original cost estimate and how they totaled up the $5.15 billion.
We talked about that.
And that was the very original cost estimate done on a cost analyst chart.
There were no personal computers at the time.
In fact, I found that in my files.
That's a very old yellow chart.
It's hard to believe some of those numbers.
On the other hand, I had a gentleman who worked for me who got his PhD at the University of Colorado and his subject was the cost of the shuttle Orbiter.
And it turns out that if we had those two years of inflation we really would have made that cost on the $5.15 in 1971 dollars.
It only ran over by about 10%.
That's right.
Let's now talk a little bit about configuration.
I don't know if you can see this chart or not.
Now, you might say how did you go from that conception in August of 1972 to something with all these numbers on it?
Now, this is really a case in systems engineering.
And let me explain to you what I mean by that.
Certain assumptions had been made.
One assumption was that in the Orbiter it was going to weigh about 175,000 pounds without payload and you were going to have a 65,000 pound payload.
You had to make that assumption.
You also had to make an assumption that you were going to use a liquid oxygen liquid hydrogen engine.
And why did you select that?
Today I'm not quite sure it was a good decision, but we did.
Why? Because of specific impulse, the ISP or the performance of the engine was the highest for the liquid oxygen liquid hydrogen chemical compulsion that we know today.
So we selected that.
Well, when you use the equations of motion, you integrate the equations of motion or any other techniques you may want to use, you then show how much propellant you're going to need to get that into orbit.
Then you know that liquid hydrogen has a density of about 4 pounds per cubic foot, very low density.
That means this big external tank is mostly hydrogen.
It's a very, very big volume.
And liquid oxygen is about 70 pounds per cubic foot.
That basically sizes your external tank.
It's a very simplified approach but that systems engineering.
You get much iteration because there is an old adage that the devil is in the details.
So you keep iterating on that with this expert team.
You then say, well, that's still not going to be the most efficient way to get you to orbit.
You say you need really a stage system, so you put the solid rocket boosters on.
You don't have what they call a single-stage-to-orbit, you really have more like a two-staged orbit and you size the solid rocket boosters and determine when they have to come off.
Basically, that is how you go about doing the systems approach using systems engineering to come up with a configuration.
Now, that is a very over-simplified way of doing it, the statement I made, but that's basically how you do it.
And these are some of the dimensions that then come out of the vehicle.
And I think they're in that handout we gave you also.
And it looks like I am going to take off.
I did know that this chart tries to lift off on me.
I don't know what the best way to do it is.
You can find those dimensions, I believe, in that paper that was handed out.
But this shows you all the dimensions of the Space Shuttle system, the solid rocket boosters, the external tank and the Orbiter.
Now, an interesting sideline in this, which I got to thinking about after the Columbia accident where the foam came off.
Of course, the reason why we put foam on was because with liquid oxygen, liquid hydrogen, at those temperatures you are going to have a lot of ice form.
And when ice forms and comes down and hits the Orbiter with the thermal protection system, I am going to talk about that in more detail, you are going to do some damage.
So one solution would be to put foam on the tank to eliminate the ice.
And, of course, you would assume that foam could stay on the tank.
Another solution would have been to not go with the liquid oxygen, liquid hydrogen engine and go with what we call storables.
Of course, they wouldn't have gotten as much performance and you probably couldn't put 65,000 pounds of payload into orbit.
But the point of bringing this to you, those are some of the decisions, as you go into various projects, you need to challenge the requirements because the requirements are really going to decide what kind of system you are going to have.
That's really the point I wanted to make.
Are there any questions?
Yes. [AUDIENCE QUESTION] That's a good question.
One thought at one time was to put the liquid hydrogen tank in the Orbiter and that would basically isolate it.
You'd put it inside.
Or, put insulation on the inside, as you pointed out.
Those were thought about.
The decision, obviously, was not to do it.
I don't recall the reason why.
But, when J.R.
Thomas comes, I will ask him that question.
That is a very good question.
Yes, it was two things.
Could you put some of this propellant inside the tank?
And the other is could you put insulation inside the tank itself?
And that was looked at.
And I guess it was complicated and very costly to do it that way, but it was looked at.
That's a very good question.
By the way, these are very good questions.
One thing, when you look at this external tank, only the very upper part is for oxygen because the density of hydrogen is so low.
I mean all of this part of the tank is for hydrogen.
So, to have put all the hydrogen inside the Orbiter, you would have a very different looking Orbiter.
You wouldn't have been able to do it.
But she really said could you put insulation inside the tank [OVERLAPPING VOICES].
Any other questions?
But that's basically how the system evolved.
Now, I oversimplified it to quite an extent but you have to realize there were a lot of iterations.
One other key thing, though, when you have new systems engineering, you do it in a team.
You usually have different capabilities on the team, arrows, mechanical engineers, electrical engineers, so you have different types of disciplines on the systems engineering team that can help you do that.
And then you have a systems engineer that sort of heads it up.
But we're going to concentrate more on the Orbiter.
And we have one of the speakers who is going to concentrate on the engine and so forth.
That's what the system looked like.
The other thing that is interesting, I think, is what the mission profile essentially looked like.
And this happens to be for STS-5, but this is the basic mission profile.
Now, before I start that, let me say this gentleman right here has flown that profile five times.
And so he's done that five times.
He can talk much more about it than I can.
One time, I will tell the story, I'm just about tired of hearing it, but when I was Director of the Johnson Space Center we had a lot of visitors.
One time I had Mr.
James Baker, who at that time was Secretary of the State, and Eduard Shevardnadze from Russia who had the same position in Russia at the time.
And I took them over to Mission Control as a visit and put Mr.
Shevardnadze down in the flight controller seat and was going to let him talk to the crew.
Not knowing that Jeff Hoffman was on duty at the time, Edward Shevardnadze spoke in Russian up to the crew.
And, before the interpreter could answer it, down comes this beautiful answer in Russian from Jeff Hoffman.
So that really floored both Mr.
Baker and Edward Shevardnadze, and me.
That was an interesting story.
Here gives you a little profile of the Shuttle mission.
You lift off.
You've reached max dynamic pressure in about one minute.
About 3800 feet is where you reach dynamic pressure.
You have the SRB sep in about two minutes.
You remember in the Challenger action, I believe it happened at about 60 seconds.
But in two minutes you get SRB sep.
And it lands the SRB by parachutes.
You have MECO, which is main engine cutoff.
The main engine cuts off.
And that, to me, is the biggest issue.
When that main engine has to burn for over eight minutes that is taking all the propellant out of that big tank, the liquid oxygen, liquid hydrogen firing the main engines.
What's the velocity of the main engine cutoff, do you know?
It's within a few hundred feet per second of [OVERLAPPING VOICES].
And then you get external tank separation.
The external tank comes down about 9000 miles downrange and lands in the Indian Ocean.
Then you use the orbital maneuvering system, the OMS engine, the pods on the back of the Orbiter.
You use that for a very short period of time to get into orbit.
Then you have your operations, whatever you're going to do, go out and service the Hubble or whatever.
You de-orbit with the orbital maneuvering system engine.
You have entry interface at 400,000 feet.
And why do you pick 400,000 feet?
Because that's where you start sensing gravity.
You get about 0.05 Gs at 400,000 feet.
And then you reenter and land either at Edwards or Kennedy.
[AUDIENCE QUESTION].
Yes? [AUDIENCE QUESTION] Why does it turn?
Yes.
You mean liftoff?
Yes.
Well, that's a very good question.
Let's see if I can repeat that very accurately.
The fact is that we used the Apollo launch pad and the ditches for the flame bucket, where the engine goes.
So, in order to get it to the right alttitude, we had to make that maneuver.
You had to make that roll maneuver during liftoff.
Is that what you're talking about, the roll maneuver during liftoff?
Yes.
We had to make that roll maneuver because we weren't in the right orientation.
We had to make the roll maneuver because it wasn't oriented correctly on the pad.
Now, let me answer your question.
The Buron was a Russian vehicle.
Well, Dan Brandenstein who was an astronaut headed the Astronaut Office saw that the Russians made this roll maneuver.
And he asked the Russians why they made that roll maneuver?
I mean they didn't have to do it, he said, because you do.
[LAUGHTER] That's true.
Did you hear that story?
Did I say it correctly?
I think so.
He said because you do.
That is a good question.
There may be one other aspect to it, and that's the question of why does the Shuttle actually fly upside down on the way up?
And I believe the original decision was aerodynamics because the thrust is asymmetric.
You have the external tank, and the Shuttle is sitting on the external tank, so the thrust actually has to be through the center of mass of the whole system.
So it is actually flying not straight but is a little bit screwed.
And, for aerodynamic purposes, I guess they figured there was less stress.
Although, recently they've started partway through the launch doing another roll maneuver.
And I think that's for communications.
I'm not 100% sure when they started doing that again.
I'm not sure either.
But the early part of the launch, when you're riding the solids aerodynamically, you go through max Q you're in much better shape if you're upside down.
Yes?
[AUDIENCE QUESTION] Well, to use a big solid rocket booster, it's not very efficient.
Many people have studied single-stage-to-orbits.
Let me just ask a question.
I know we've got a mixture of aero-astro and TPP, ESD.
Are there people here who have not seen the rocket equation?
Everybody.
Well, OK.
That's very important.
Let me just very briefly If you have a rocket and you want to increase the velocity of the rocket, I mean that's a critical thing, by a certain delta V, you can take the mass of the rocket when you start the burn, which we call M sub i, the initial mass, and the final mass.
And, of course, the initial mass equals the final mass plus the mass of the propellant.
And that equals an exponential to the negative power of the velocity decrement, which you're trying to put in, divided by the exhaust velocity of the propellant that's coming out.
Now, this is usually phrased in terms of what we call the specific impulse times gravity because it's done by engineers.
That's right.
So you will hear reference to the specific impulse and the units of that are seconds.
But, if you multiply that by the gravitational acceleration, it will actually give you the exhaust velocity.
Because this is in an exponential it is extremely sensitive to the exhaust velocity.
Whatever delta V you are trying to get out, if you can add a few more seconds to the specific impulse then the actual mass of propellant that you have to burn in order to create that delta V goes down substantially.
Now, for hydrogen/oxygen, the specific impulse is about 450 seconds.
For solid rocket motors, the specific impulse is on the order of about 250, something like
that. That's what I thought it was, about 250.
That's almost a factor of two.
And you put that into an exponent, if you try to get into orbit with purely a solid rocket motor, you need a lot more propellant.
And that means the payload mass you can take into orbit is reduced.
That's why, in addition to using hydrogen and oxygen, and we will have a lecture specifically on the main engine, they got every last ounce of performance out of it by running it.
You'll hear references to running it at 104% abraded thrust, 109% abraded thrust.
And all of these have engineering consequences because, in order to increase the exhaust velocity -- Which, of course, if you do that, that's in the denominator so that's going in the right direction, you have to increase the chamber pressure, the temperature and all of the design problems.
He is going to ask you the question why don't you use liquids all the way?
I knew what your question was.
[AUDIENCE QUESTION] He is asking why don't you use the liquid hydrogen engine all the way rather than using solid rocket boosters?
Well, first of all, why not just have a bigger fuel tank, one big fuel tank and just use the Shuttle engine?
That's what he's saying.
That would be essentially single-stage-to-orbit.
And I'm not going to spend a lot of time with the rocket equation, but the delta V that you need to get into orbit is, well, orbital velocity is just under 8 kilometers per second.
But to that you have to add the gravity loss [OVERLAPPING VOICES].
And so the overall delta V is effectively about 9 kilometers per second.
If you put that into the equation, you actually can get the ratio of the final to the initial masses.
And even for a hydrogen/oxygen engine you can basically almost 95% of the mass sitting on the launch pad has to be propellant.
Which means that all of your structure can only be about 5%.
Now, if we could make super strong, super light structures, like an eggshell, then, in principle, we could get to orbit without staging.
Now, the first thing you learn in Rockets 101 is why you cannot do single-stage-to-orbit.
It is because right now we do not have the technology to do that.
Well, see, many people have studied single-stage-to-orbit.
In fact, NASA tried to build one the X-23 and we failed.
What they say is really what you need is to be as smart or as high-tech as an eggshell, be able to make the structure as thin as an eggshell, hold all the fluid in it and have very low density.
Some day maybe we will do it.
[AUDIENCE QUESTION] Basically, by using the boosters or, in a more traditional rocket, a first stage, once you've exhausted the fuel then you drop all of that structural mass.
And so, for the second stage, this initial mass becomes much lower.
[OVERLAPPING VOICES] The other thing that's interesting is that if you go back to Apollo, during Apollo there were three ways to go to the moon.
One was direct.
Von Braun wanted to build a large vehicle called the Nova where you actually lifted off from the Cape and sent the whole vehicle right to the moon.
The other was to do earth orbital rendezvous.
And the other way was to do Lunar orbital rendezvous like we did.
It turns out that that Nova vehicle had to be so big that that was ruled out, so we wound up with earth orbital rendezvous.
Yes, sir.
How much did the role of geography play in the design considerations?
Because just looking at that, I mean, you have to have the external tank separate at a specific [OVERLAPPING VOICES] talking about launching in Vandenberg.
Originally we thought about launching out of New Mexico.
Actually, when you're doing flight design, depending on whether you're going into a due east launch to go into a 28 degree orbit or a high inclination orbit, the placement of the external tank reentry is a major factor in flight design.
We spent a lot of time on that.
And, in fact, often the trajectory has to be shaped to be not quite efficient as it otherwise might be because you have to control the landing of the external tank.
But your question is a good one.
I did some consulting for a company, after I retired, and we were looking at a commercial launch vehicle.
And we were trying to launch the vehicle out of various places in the United States.
And it's very difficult to get approval because of the concerns they had for types of failures or anything that you're going to do.
Yes, sir.
I'm not sure why I thought this, but I was under the impression that the external tank burned up in the atmosphere.
No. It didn't hit down. It breaks up in the atmosphere.
It didn't come down as one big glob.
Some of it burns up, but some of it does get back to the ground.
OK.
Is there any talk about a need to clean up all that?
Well, they haven't yet.
I'm not an astronaut.
That's mainly aluminum.
Remember, the liquid hydrogen, liquid oxygen inside evaporate and are non-toxic.
They question that Dr.
Young asked about the solid rocket booster environmental problem is a real problem that had to be solved.
The other problem I had, I worked on trying to get the Galileo cleared because it had a radio thermal nuclear generator on it.
To get that cleared going into orbit is a big job.
I worked on that and thought I would never finish it, but we finally got it cleared.
But it was a very tough job.
We had RTGs cleared to be launched.
I don't remember specifically all the noxious chemicals that come out of the solid rocket boosters, but it is pretty nasty stuff.
Yeah, it is.
I remember one of my launched, the families and guests are taken to a launch site viewing area about three miles inland from the launch pad.
And it just so happened that the wind was blowing onshore that day, it was an afternoon launch, and my brother is a real space nut who always liked to watch as the rocket went.
After about seven and a half minutes it just sort of disappears over the horizon.
But, after about five minutes, the solid rocket exhaust was actually approaching the spectator area because of the wind blowing in.
And they made everyone get on the buses and drove them away so that nobody got injured.
And my brother was really annoyed because he didn't want to leave.
If we have time, I would like to talk to you a little bit about the Challenger accident, talk to you about the O ring seal.
I would like to talk to you about that.
I don't want to sweep those things under the rug, but I would like to talk to you about that because I've got my own thoughts on the problem that I would like you to hear.
OK.
Any other questions on the mission profile?
Shall we keep going?
Yeah.
Tell you what.
Let's take a two-minute break, stand up and turn around.
An hour is a long enough time to sit for a bit.
I am assuming that everybody is aware that the Russians, at one time, had a space Shuttle program.
And they manufactured a vehicle which looked almost identical.
Not exactly but almost identical.
And it was no accident because they used our plans.
They used our thermal protection system, too.
And they only ended up flying it once.
And they actually flew it unmanned.
And, despite a little bit of nail-biting during the landing, they did recover it successfully.
And then they discovered, just what we had discovered, that it was a lot more expensive to operate than they had anticipated.
And they had a lot less money than we did.
And so it basically never flew again.
They had crews of cosmonauts who had been training to fly the Buran, but there were actually two differences.
The first was that they put the engines on the external tank.
This did two things.
First of all, it did improve the performance.
And also the Russians were turning out engines on assembly lines basically.
They turn out a tremendous amount of rocket engines.
And I guess, from their point of view, and I don't know the details of the performance of their engine, how their main engine is compared to ours, I think they actually had four engines, if I remember.
[AUDIENCE QUESTION] The Orbiter was just a glider, and it was no difference.
It was an unpowered glider.
They did learn one thing from us that when you have a delta wing vehicle you are very, very sensitive to the center of mass of the system.
And it was a problem because the Orbiter, when it flies back through the atmosphere, it hits the top of the atmosphere at mach 25 and then flies all the way down to subsonic.
And the control characteristics and stability characteristics change throughout that flight envelope.
And we will have a lecture specifically on the aerodynamics of the Shuttle, but it turns out that the Shuttle is extremely sensitive to the forward CG.
And I think it was around the mach 3 flight regime, if you're just an inch or two forward of the critical area in the CG, you can lose control.
And so we actually, on many flights, they always do a weight and balance on the Shuttle before a launch, have had to be put lead ballast in the aft engine compartment of the Shuttle just to get the CG far enough back to get mach 3 stability.
I hate to tell you how many tons of lead we've launched into orbit over the course of the Shuttle program because of the CG.
And, if you look, the delta wing profile of Buran is slight different from the Orbiter because I guess they learned the lesson.
And so they were not as sensitive to the CG.
But, of course, once you build an Orbiter you cannot really change it.
And so we were sort of stuck with it.
To add on, one of the other concerns was having the Orbiter with the thrust behind the Orbiter and this large mass in the tank you could get a pogo-type activity.
And so one concept that Max Faget had early in the program was to have a swing engine, actually have the engines fire on the back of the tank and then, when you get ready to separate the tanks, swing the engines back into the Orbiter and bring them back into the Orbiter.
And we threw that out.
That was a little complicated.
You're going to have a discussion on mechanism in mechanical systems.
And, mechanisms in mechanical systems, everybody can design but everybody has a hard time making them work.
When you put up an electrical schematic everybody accepts it.
When you put up a mechanical drawing of a mechanical system everybody has an idea of how it is supposed to work.
Let's move on.
I've got some pictures now just to show you.
It is showing a profile.
And then, of course, you've seen the vehicle when it gets to roll out of the assembly building.
There it is stacked.
And then it's on a crawler.
This crawler basically was used for Apollo, and the crawler takes the vehicle out the pad.
Just one thing so that people notice the difference here.
Is anyone familiar enough with Apollo to be able to say what the fundamental difference is with the crawler mechanism here and the crawler in the pad?
Well, if you look at the picture of a Saturn rocket being rolled on the crawler, the whole launch tower was on the crawler.
And so they rolled the whole thing out.
With the Shuttle it's a little different.
I will be bringing in some pictures at some point to show you some of these details, but they actually cut off the top part of the Saturn launch tower because the shuttle stack isn't quite as tall.
And they added a movable what's called a payload change-out fixture.
And so once the shuttle actually gets on the pad, there are actually railroad tracks here.
Is this a better picture of it?
OK.
Yeah.
This whole enclosure rolls over and forms a kind of hermetic seal around the shuttle so that you can open the cargo bay doors which, by the way, cannot support their own weight in one G.
So you have to put an external strong back on them.
And, in the meantime, you put the payload that is going to be installed, this payload canister here, that is installed in the change-out room.
And then it is swung over, the doors are open, the payload is installed in the bay.
And then this forms a protective enclosure over the shuttle.
And it is not swung back usually until the day before launch.
See, now this is again another detail in systems engineering.
You go in for that schematic or that little plan form of the Orbiter, and you did iterations of the size and then you had to do iterations of the launch complex, how it was going to put payloads in.
And that's all systems engineering, certain levels of systems engineering.
I mean it's very easy to say, well, we will just put the payload in on the pad.
But the actual development of the mechanisms to do that is extremely complicated to say nothing of which, I mean what kind of amazes me is that it has to be done essentially in a clean-room environment.
You're up there crawling around the pad inside the payload change-out room in white coats, bunny suits and gloves on.
And outside the wind is blowing and it could be raining.
There is sand blowing by.
So the whole thing has to be done in a clean-room environment, but it's on the scale of a naval shipyard.
This is a huge vehicle.
So it's really a challenge.
Of course, that's one of the reasons, in all honesty why the cost per flight has gone up.
The original concept, when Dale Myers was Associate Administrator of Manned Space Flight, the man who spoke to you, I went up to him, seeing I was the Orbiter Project Manager.
And we concluded this will be very simple.
We were going to make very standard payloads.
You went, put the payload in, fixed it in, we were going to launch it, deploy it, come back, fail the computer on the pad, we'd go anyway, wouldn't replace the computer.
Of course, that all changed.
The missions became very complicated and we didn't do that.
That is another important point, though.
You better be sure, when you get to be a project manager or manager, you understand your requirements, understand your customer's need.
But, if the ground rules change, your performance is going to change.
So you need to understand that.
Any other point you want to make, Jeff?
Any other questions?
I've just got some other pictures.
Here is the liftoff, as you see, everything burning.
Have we had a case where we hit a bird on launch?
Yes, I believe we have.
Of course, in the Orbiter windows, one of the things we did, I hate to say this, is fired dead chickens into the window to see if you could break the window in the system.
I think we did an aircraft also.
And we actually did, I remember, one simulation where in the simulator, right at liftoff, the instructor came in and threw a rubber bird in the commander's lap and said you've just been incapacitated by a bird strike.
And so the copilot had to take over flying.
You should realize that Kennedy Space Center is actually a wildlife sanctuary, and so there are a lot of birds.
There's a National Wildlife Sanctuary, park rangers and a lot of eagles.
There are eagles and a lot of turkey buzzards.
There is a landing strip that is right in the middle of the bird sanctuary.
And so often, before the shuttle gets ready to come in, they will send planes to sort of buzz the runway to scare the birds away.
They also had a problem at one point with owls.
No, woodpeckers, excuse me.
Woodpeckers were decided that the external tank insulation was a good place to find bugs.
It sounds funny, but think of what that actually potentially could mean.
They had to go down and had loudspeakers and stuffed owls and everything, which they ended up putting around to scare away the woodpeckers.
These are things, again, which I think the original systems engineering never took into account.
We were worried about F equals MA.
And there is a typical deployment of [OBSCURED], if I'm not mistaken, to get the payload into orbit.
Here is a picture of the crew at their station with their CRTs.
And, of course, then you de-orbit.
And this is, of course, not a real picture.
Do I have that right?
This is right.
And then, of course, landing on the runway.
That's a typical profile that we've gone through in terms of showing.
Now, let me talk a little bit more about systems engineering.
When I took over in August of 1972, we had what we called Space Shuttle Orbiter Management Reviews.
That's North American.
This was one of September 1972.
And I think it might be interesting to look at the agenda in September of 1972 what we were talking about.
And here was the agenda.
I'm not going to go through all the paperwork but here was the agenda.
Basic resizing of the vehicle because of certain problems we had in the iteration.
Cabin configuration and docking location.
The Space Shuttle main engine interface control document.
It turns out interface control documents are probably one of the most systems integration tool because interface control document defines how you're going to put the main engine into the Orbiter.
What is interface?
An interface could be this plug.
If you had a plug in a socket, it would be bad if you couldn't plug that in.
But that's an interface.
When you change your tire, if those bolt hole patterns don't match you'd be very frustrated.
So that's an interface.
Interfaces can be as simple as that or they can be very, very complicated.
And so this was the SSME interface control document, how we actually get the main engine in terms of mechanical propulsion put into the Orbiter.
Integrated asset control, abort requirements.
And now we're talking about just procurement, RCS and OMS procurement.
The rocket motor's procurement package.
Avionics definition, leading edge TPS and emergency risk.
These were some of the problems we started talking about.
Now, these are lower level systems engineering problems.
But they are systems engineering to make the definition of the total launch configuration.
Here is an interesting chart.
This is dollars.
Why this is interesting is you see on this chart Rockwell's proposal.
These are $1 million.
$123 million the first year, but when I became Orbiter Project Manager that was Rockwell's proposal.
The first thing they did to me, they told me I only had $73 million that year, so I had to re-phase the whole program.
I had to rephrase the whole program to fit the funding curve that the Office of Management Budget gave me.
This was a major perturbation.
When you do that and you don't get your money as the proposal, it comes out to be a cost increase.
Right away we went a little bit cost increase to 4.6, but as you don't get the funding requirements you then essentially lose your momentum, you lose your schedule.
And once you lose schedule you start costing.
As Dr.
Hoffman mentioned, you have the three-ledged stool, cost, schedule and performance.
And that is a continual tradeoff as you go through a systems engineering program.
And the things you have to look at, the things that cause project managers to lose their job is first you're going to recognize that you have a weight increase in the vehicle.
Whatever you build is going to cause to have your weight increase.
The next thing you're going to have is a schedule slip.
And then, when you put those together, you're going to have a cost increase.
And then you're going to have technical problems.
And those are disastrous cases for a project manager.
Those are reasons to firing somebody.
Luckily I made it.
I made it because of a man that you are going to hear talk, Chris Kraft, because he was my immediate boss and he ran interference for me.
And you're going to hear Dr.
Kraft talk.
He's a good man to have on your side running interference for you.
You're going to hear him talk.
He's a great guy.
And he's going to tell you what he thinks.
And he'll tell you what he thinks.
But that's a little bit about cost.
Further systems engineering questions in the Orbiter Management Review is Orbiter maximum wing size.
ATP is our authority to proceed.
Wing size is very important.
You've got to get the aerodynamic model wing load squared away.
We said no major line change after program requirement is reviewed.
Controlled dry weight was 170,000 pounds including margin.
And I don't think we made that, did we?
No, we didn't.
More like 200,000.
No ejection seats on vertical flights.
Top located docking port.
Wing area for landing speeds of 150 knots.
Wing stiffness criteria.
Flutter margins.
Control effectiveness for entry and ferry.
Elevon design concepts.
[OBSCURED] crew cabin.
These were some of the systems engineering problems that had to be resolved before you could start manufacturing.
One of the interesting things, I forgot who was head of the astronaut office at the time.
I don't know if it was Brandon Stein.
I don't know who it was.
At which time?
Well, at about the time of the design of the Shuttle.
About this time.
[AUDIENCE QUESTION] I forgot who I asked, but we were trying to get the cockpit laid out.
It was very important to get the cockpit laid out.
And I wanted the crew to have their inputs on how they wanted the cockpit.
You want the crew to be a part of laying out the cockpit.
I called the head of the Astronaut Office over and said we need to lay out this cockpit and you need to come back to me with a decision of how you want the cockpit.
He said I cannot do it.
I've got 100 astronauts over there and they won't agree.
I said I'll tell you what you do.
You tell them that I will give two weeks and then I'm going to do it.
And that really scared them because I didn't know anything about laying out a cockpit.
And we had the cockpit laid out.
So that's sometimes how you have to use management techniques.
Now we're going to get more into the details of what you're going to hear in some detail.
And I'm going to start if off a little bit.
Here are basically the hardware subsystems.
You're going to hear a technical briefing on the thermal protection system and the structures.
You will see in your syllabus when that is, but you're going to hear a very detailed discussion of the thermal protection system, the structures by Tom Moser who actually did the work.
He was actually what I call my subsystem manager on the Shuttle in the early days of the program.
You are going to hear a technical briefing on the Space Shuttle main engines and a very detailed briefly by J.R.
Thompson.
J.R.
Thompson, a little background about him, he was Project Manager of the main engine at Marshall Space Flight Center.
Then he became Director of the Marshall Space Flight Center.
Then he was Deputy Administrator at NASA.
Now he is Vice President of Orbital Sciences.
You are going to have a detailed briefing on the hydraulic system, the auxiliary power system, fuel cells, orbital maneuvering system and the reaction control systems.
That is going to be by Henry Pohl.
Guidance, navigation and control is going to be by Phil Hattis from the Draper Laboratories.
Environment control and life support of the crew cabin, that is going to be a man who is currently working at the Johnson Space Center, Walt Guy who actually did this job for the Apollo.
Most of these people, by the way, did the same thing for the Apollo that they did for Shuttle.
Very similar jobs, so you can ask these people about the Apollo program.
Landing and mechanical systems by Al Louviere.
Communications and electrical power we're really not going to brief, although we will talk electrical power when we talk fuel cells, but we're not going to really have a discussion on communications and fuel cells.
And then we're going to have some analytical studies on aerodynamics and aerothermodynamics.
These will probably be detailed technical discussions when we get into aerodynamics and aerothermodynamics.
Again, these people did the same on Apollo as they did on Shuttle.
That is going to be your details of what you're going to see.
What I'd like to do now, with the few minutes we have remaining, is talk to you a little bit and give you a little bit of flavor of some of these subsystems, a little bit more detail than that we've previously given you, but not as much detail as you're going to see.
Let's start off a little bit with the Orbiter and just show you what the Orbiter looks like.
And talk a little bit about some of the subsystems in the Orbiter.
The forward reaction control systems is up in the far nose of the vehicle.
And then you have the reaction control system back in what is called the OMS pod, orbital maneuvering system pod.
You have reaction control systems here and here.
That's reaction control systems.
You have your Orbiter maneuvering system back in these OMS pods.
Of course, you have the main landing gear and the nose landing gear.
The wing, faring and the leading edge, which is the carbon material.
This wing edge is carbon material.
And the side hatch.
And the overhead windows.
And then the side windows and the far windows.
The payload bay doors are made out of graphite epoxy.
They were originally made out of aluminum.
We made it out of graphite epoxy because of the weight growth.
We could say quite a bit of weight if we went from aluminum to graphite epoxy.
That was the largest, probably at that time, composite material used in an airplane.
It was made out of graphite epoxy.
And then the main engines, of course, are in the aft end of the Orbiter.
And then you have the vertical tail stabilizer.
And, of course, you've got your elevons.
And you've got the body flap.
The body flap was actually put on originally to deflect some of the heat from the engine, but it actually turned out to be a control surface also.
It actually turned out to be both the focus of a control surface.
That's sort of the layout.
And you can visualize where you use these systems, these active systems.
The reaction control system is used primarily in orbit getting ready to de-orbit.
The OMS is used to help you get into orbit and get out of orbit or maneuvering you want to do.
The body flaps and the elevons are actually used primarily during entry, a little big during assent, during entry.
We do use the RCS system a little bit during entry.
In the high altitude of entry you use the RCS system, but eventually during entry the load just becomes so high the RCS system or reaction control system does not become very effective.
I guess that's about all I want to say.
Any questions on that?
Yes, sir.
I was wondering why the nose gear was so short.
Well, it actually saves weight making it shorter.
It was easier to put in and it saved weight.
And I think also when you land, by having the nose down you actually have an aerodynamic force pushing the Orbiter down on the runway which increases your stability.
And since you don't have to worry about eventually taking off.
Like Professor Cohen said, if they had wanted the Shuttle to be able to take off horizontally from the runway they would have had to raise the nose.
Yes, sir.
[AUDIENCE QUESTION] Well, when you say under-designed, I mean I would call it a minimum design.
I mean if it were under-designed it would break.
I mean there are landing limits.
As I said, for a planned nominal landing, and you have to realize there are different kinds, there are emergency limits and limits for nominal operations, you can take off with 65,000 pounds.
That means if you have a launch abort and you have to return and land with your payload, you will be landing with 65,000 pounds.
But you don't plan to do that.
That's a one off deal.
If you're planning to do it, it means you can do it over and over again and then the limit is 35,000 pounds.
They don't build it like a Navy carrier landing airplane, I will tell you that.
Not just landing gear, I found the tires are just really small.
The tires are small.
They have no treads either.
And the brakes were small.
Actually, the original brakes were under-designed and we kept having brake failures.
In fact, I took the drag shoots off and then we put them back on.
I took them off because of weight and put them back on.
Tires are very interesting.
We're going to have a very detailed discussion on tires, but tires are very, very complicated.
You think by putting more plies on the tires you make it stronger, but not necessarily because when you cycle them they got hot and the more plies tend to hold the heat in and weakens the tire.
Tires are not a very simple thing to design.
They are only used once then.
No.
I think we used these tires, I think, five times, or could use them up to five times.
The brakes are used five times.
I don't know about the tires.
I don't know.
Let me talk about weight and margin.
Let me go back to Apollo.
We had Apollo designed.
And we found that on the Lunar module taking off from the Lunar surface it was too heavy.
We couldn't lift off.
The engines were already built, the tanks were already sized and we couldn't get off the Lunar surface.
And that's not a very good deal.
The astronauts didn't like that too well.
George Low was the program manager at the time.
We were spending, in those year dollars, $24,000 a pound to get weight out of the Lunar module.
And they scraped, they did everything they could to get any [OVERLAPPING VOICES].
You're basically offering them $24,000 for every pound that they could scrub out because we couldn't resize the engine.
The engine was there.
They were literally in there with emery cloth shaving down the aluminum from the inside.
So weight can be very critical.
I don't think most people realize how close the margins were there.
I mean the Lunar module was only certified for, I think, five pressurizations.
And then it was beyond its structure limits.
My statement to many people is the next time we go to the moon we are going to find out how hard it really was because, to tell you the truth, we went to the moon with one computer in the service module and one computer in the Lunar module.
Excuse me.
We did have two computers in the Lunar module.
We had the MIT computer and then we had a strap-down system built by TRW.
That's an interesting point.
I think somebody asked the question last time.
Let me talk about the aft end of the Orbiter a little bit because we did not have CAD/CAM systems at the time.
And let me show you some pictures taken.
I don't know what vehicle it is, but that's the aft end of the Orbiter.
That's where the big engines go into.
This looks like it is going to take off.
If you crawled in the back of the aft end of the Orbiter there is plumbing, there is wiring, there is structure.
Now, had we had computer-aided design at that time, I am convinced we would have a much neater, better design than we have today.
We had to mark everything up.
We marked it up and we changed it.
People bumped their heads on it.
If you go to the aft end of the Orbiter you'd wonder how they can do anything, but that was done because we did not have computer-aided design.
Like airplanes now have virtual markups.
You can actually build a virtual markup right on your computer.
We didn't have any of that.
And this, again, is just to show you what the crew module looks like in a structural point of view.
That was the pressure vessel.
That's basically a pressure vessel going together.
And there were the windows.
But the thing that's interesting about that, it had very intricate welding techniques.
And these techniques were very good and actually proved to be a very, very satisfactory design in a pressure vessel.
I would like to now talk a little bit about a couple of subsystems that you're going to hear much more detail about.
But now venture into some systems and tell you a little bit about them.
It's probably going to be the thermal protection system and the guidance and navigation system.
I will talk to you a little bit about that.
Here is an old chart that shows, now, unfortunately I use English units, maximum heat rate and BTUs per foot second squared, integrated heat load and radiative equilibrium temperature.
And then you see the Apollo return as number four.
And the Apollo design was about 100 BTUs per foot squared integrated heat load, if I read the chart right, and a heat flux of about 300 BTUs per foot squared second with a radiative equilibrium temperature of about a little over 5000 degrees Fahrenheit.
On Apollo that was our baseline.
We used an ablative head shield.
Now, the ablative heat shield has a density of about 130 to 140 pounds per cubic foot and is not reusable.
On the Shuttle it was about 40,000 BTUs per foot squared integrated heat load and 50 BTUs per foot squared second for heat flux with a radiative equilibrium temperature of about 3000 degrees Fahrenheit.
Now, at that point of time we had to come down from our baseline knowledge of an ablative material to what we could use and make it reusable and light.
And there were several competitors at the time.
One was a metal insulation, Rene-41, I believe, shingle-type material, very thin, very high temperature, and it had pretty low density.
And then, of course, there was the ceramic material or the so-called tiles which was basically a ceramic material.
It is made out of silica.
In fact, interesting enough, it's made out of what we call gopher sand that comes from Minnesota.
Why is it gopher sand?
I'm not quite sure, but it's got a very high pure silica content.
And you make it into a mulch and then you actually bake it and it comes out in these tiles.
And, of course, tiles sometimes are hard to bond to the vehicle.
How many people knew the tiles were hard to stay on the vehicle?
Well, I was the Orbiter Project Manager and my home town is San Antonio, Texas.
We would go back to San Antonio to visit my family.
My little old aunts would say their nephew is in charge of the shuttle program and he was having trouble making the tiles stay on the vehicle.
They couldn't understand that because they had tiles in their kitchen and their bathroom and had no trouble at all having those tiles stay.
So they couldn't understand why I was having so much trouble having the tiles stay on the vehicle.
We had a problem.
What would we use?
There were two competitions on it.
One was the competition from two of the research centers.
The Langley Research Center wanted to go with the metallic version and the Ames Research Center wanted to go with the ceramic version.
And at JSC we're trying to figure out which one to go with, and we decided to go with the ceramic version.
Of course, Tom Moser is going to give us some detail because Tom was the guy really in charge of it.
But just to give you a little background, and he'll go into more details of it, the problem we had with the tiles is that when you bonded the tiles, of course, they were basically a ceramic material.
Forget about this layer called densified for a moment.
When you bonded the tiles to the room temperature vulcanizing material, which is basically a high temperature glue, to the strained isolator pad, you got stress risers set up and the tile became very weak.
It broke really at the bond line of the tile because it became very weak.
The stress risers were such that because it was ceramic it broke at the interface.
We had one very cleaver engineer that saved my day because tiles were falling off and I couldn't get the vehicle built.
He said if we densified below a quarter of an inch of the tiles, and tiles could be anywhere from two inches to four inches thick, and by that I mean put liquid glass inside that tile, the bottom became like really a hard surface, no stress riser, you could bond it like this and basically have the inherent strength of the tile.
It no longer broke at the bond line, it broke at the tile, which had a very high load.
So, after we did that, we really did solve the tile problem to a large extent.
That was the day we really solved the tile problem, but I remember this so distinctly that we were doing all these tests and all these analyses and all of a sudden they called me one day and said Aaron, we're just doing a very simple flat-wise tension test and tiles were just coming off the vehicle.
So that is what saved the day.
I think it's another good example of problems in system engineering if you just leave it there for a moment.
Sure.
We worked so hard figuring out how to make these tiles, and they are extraordinary.
You can take one of these tiles, put one side of it into a kiln, heat it red hot and you can hold the other side of it in your fingers.
I mean that's how good the insulation is.
And I guess everybody just sort of figured we know how to glue things on.
You brought up another very good point that I left out.
The problem is we spent so much time figuring out the efficiency of the tiles to do the thermal protection.
In other words, this is fantastic tile.
It weighs about like balsawood, so it is anywhere from 10 to 12 pounds per cubic foot compared to 130 pounds per cubic foot.
It can take the higher temperature ablative material and be reused.
So it answered all the questions we had about thermal protection.
What we didn't do, though, was figure out how we're going to attach it to the vehicle.
But everybody, I think, just assumed, I mean it's almost like your relatives, we glue tiles in the bathroom all the time.
Anybody can glue stuff onto something else.
But we really did a bad job.
In fact, that's an example I use in my course at A&M.
We come up with a function structure.
What functions does this have to perform?
Well, it doesn't actually have to thermally protect the vehicle.
It has got to stay on.
And we forgot about the staying on part.
We talked about the thermal protection part.
And this thing is so good, its thermal diffusivity is so good that, like Professor Hoffman says, you could get the surface temperature to 2000 degrees Fahrenheit and hold it in the palm of your hand.
And so it turned out it was a very, very good solution but we almost blew the day by not coming up with this design.
Why does it have to be individual tiles rather than big long panels?
Well, because of the coefficient expansion.
Now, that's the point of this strain isolation.
And it's another interface problem which is part of the system's engineering.
The structure of the shuttle is aluminum.
Thermally, it expands and contracts by several inches every time you go from night to day.
I mean the Orbiter, we've done these measurements.
One side of the Orbiter is pointed towards the sun.
The other side is cold.
The Orbiter actually bananas by a couple of degrees.
And they did thermal tests in some of the orbital flight tests where the payload bay, the clearances could change by a couple of inches depending on the thermal conditions and designing mechanisms to latch them closed with those tolerances.
So the orbiter is flexible and expands and contracts as a metal.
The tiles don't.
They are rigid.
And so, if you have too large a tile area, the aluminum is going to bend under it and the tiles will crack.
And even with the small tiles, between the aluminum and the tile there is this strain isolation pad.
This of it as sort of like a felt type material.
It kind of eases the interface between the two.
And, of course, if you look at the tile installation on the vehicle, in between the tiles, remember on the last mission we went EVA to fix the gap fillers between each one of those tiles mainly for problems during liftoff to keep the tiles from vibrating and damaging each other.
And those gap fillers, I'm not concerned about going EVA to fix it.
Well, on the other hand, once you see the problem you cannot let it go so you've got to do something about it.
They went EVA and pulled the gap fillers out, but those are the tile installations.
But your question is a very pertinent one.
Why are there so many?
And that's the reason, because of the coefficient expansion.
Then you put gap fillers in between those tiles to keep it from damaging during liftoff.
It does turn out that one of our biggest concerns is going to be how fragile the tiles were.
And they really turned out to be a pretty good system.
We were concerned about losing a tile and then getting heat and having an unzippering effect where you lose a bunch of tiles.
And we really alleviated that problem.
I will tell you another funny story.
This is probably not so funny but it was a story anyway.
I've forgotten what mission it was but these tiles are actually waterproof.
We actually put Scotchgard on them because the waterproofing is in the tile.
And after a certain mission the waterproofing boils out so we had to waterproof the tile.
STS-4 was the hailstorm.
That was the first one where they recognized the problem.
Well, anyway, Rockwell International was my contractor.
The head engineer at Rockwell was a brilliant guy, but he ran a test where he put a densified tile panel in a bucket of this Scotchgard or this rain repellant material.
And he called me about 11:00 at night.
And I was getting ready to go in because we were [OBSCURED].
We just ran this test and all the tiles came off.
I said what do you want me to do with that information?
We're getting ready to back the de-orbit burn.
I mean they were coming down.
I said what do you want me to do?
I said that was sort of a dumb test, wasn't it?
I said is that what happened on the vehicle?
Did you actually put the tiles in a bucket of water repellent material?
He said no.
I said that's a dumb test.
I took it upon myself, after talking to the man you're going to hear, Tom Moser, what do we do with it?
We didn't tell anybody.
Now, I took a big risk on that.
But what could you do about it?
There was absolutely nothing you could do about it on that test.
So that's an interesting case in discretionary project management.
But you will hear more.
Let me just mention the question of waterproofing because, again, that was something that I think at first slipped through the systems engineering.
And it was the fourth shuttle flight STS-4.
I was down at the Cape for some other activity.
The night before a launch they had rolled back the payload change-out room, the whole shuttle was exposed.
Well, a thunderstorm with hail came through, and it pelted the bottom of the shuttle.
There were pox marks all over it.
And, of course, it was raining.
And people realized that the tiles actually were absorbing material.
The outside of the tile is kind of impervious, but once you crack that the rain can get in.
And they actually brought a tile expert down from the Cape and did some calculations.
It was an early flight so we didn't have a maximum payload luckily because we ended up carrying, they figured, probably a few hundred, many over a thousand pounds of water into orbit.
And there was so much water in the tiles that the orbital dynamicists were able to measure the perturbation of the Shuttle's orbit because of all of the water that was evaporating.
And, of course, it was evaporating from the bottom so that it effectively was asymmetrical and had a propulsive force.
And after that was when they came up with the idea of Scotchgarding.
And, if you look closely at each of the tiles, there's a little hole where every tile before every flight they go through with a hypodermic syringe and just inject a little bit of Scotchgard.
And think how critical it is.
You've got over 30,000 tiles.
If you add one ounce to every tile, you do the math, that's a lot of extra weight.
So you want to put in as little as possible Scotchgard but enough to do the job.
This might be a very good subject for you to decide to redesign.
You might think about what are the technologies, what are the differences, how would you do it differently because their technology has changed.
That's why I brought this one up.
And you will hear a much more detailed discussion by Tom Moser, but you might think about this as one of the subsystems to look at.
I guess that's all for today.
OK.
We will see you on Thursday.