Flash and JavaScript are required for this feature.
Download the video from iTunes U or the Internet Archive.
Topics covered: Aerodynamics - (From Sub - to Hypersonic and Back)
Instructor: Guest Lecturer - Bass Redd
Subtitles are provided through the generous support of Heather Wood.
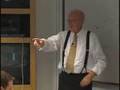
Lecture 7: Aerodynamics - (...
Related Resources
Bass Redd Bio (PDF)
A couple things.
First of all, this is for those of you who are working on GN&C for your projects.
I have spoken with Phil Hattis from Draper Lab, who is going to be giving the lecture on GN&C, but he is not giving the lecture until the third of November.
Now, that is kind of late.
And so I asked him if he would be willing to meet with all of you who are working on GN&C just so you could talk with him and ask questions.
If you want him to give you a short version of his presentation that he's going to give in November, he can do that.
But he is our in-house resident expert.
And I figured it's better if you get a chance to talk to him early.
He has some time free tomorrow and he has some time next week.
He is out of town through today.
I know we have two teams working on GN&C.
If you guys want to get together and find out if there is some time when you could all meet with Phil, I will give you his contact information.
He says he is happy to do it.
It would be much more convenient, from his point of view, if you could all see him at the same time and talk about it together.
Let me know what you come up with.
But, as I say, he is happy to do it.
Second thing.
There was an interview printed in US Today, which I have posted on the class website with Mike Griffin, the administrator.
And it says NASA administrator says space shuttle was a mistake.
And so the question was what do we intend to do about that in this class?
Well, let me actually read the specific words that Griffin uses because I think that's important.
Griffin said my opinion is that the Shuttle was a design which was extremely aggressive and just barely possible.
And that's actually, I think, a slightly different way of looking at this.
I mean I would also say that Apollo was a project that was extremely aggressive and just barely possible.
And I think that it is actually NASA's business to do things which are aggressive and just barely possible.
Now, whether it was the right decision for the country to abandon the Exploration Program after Apollo and do the Shuttle and then later on the Space Station that's more, I think, in the political realm.
And, as I think you've heard both from Dale Myers and John Logsdon, that, in fact, there were political determining factors which didn't really give NASA a lot of choice.
Just to remind you, the basic point of this course is not the political and economic history of the shuttle.
It's a technical exploration of how we did the shuttle.
I think we would all agree that the concept of the Shuttle was extremely aggressive and the technology was being stretched and it was just barely possible.
And, yet, we did it.
And the idea of this course is to understand how we did it and how the systems work.
I think it will be interesting, when we get towards the end of the course, maybe we'll sit down and relax and instead of having, I think there are two kinds of open sessions towards the end that I've been thinking about what we want to do there.
And in one of them I think we may just talk a little bit and reflect on what we've learned, and that will give us an opportunity to discuss issues like this.
That's all I'm going to say about this.
I don't propose that we discuss this further today because we really are fortunate to have Bass Redd.
And Aaron Cohen is going to introduce him formally.
I will just say that when I was at Johnston Space Center last spring and I met one of the older managers there and I was telling him about our plans for this course and how Aaron was going to be a visiting professor here and we were inviting some of the experts in the early design of the Shuttle, he said, well, there is one guy you absolutely have to get, and that's Bass Redd.
Well, we've got him.
We're very fortunate.
And, Aaron, I will turn it over to you and let you explain a little bit more why we're so fortunate.
Well, first I have to tell you how I found Bass.
I had a hard time finding Bass.
He lives in a placed called Smithville, Texas.
And, when people ask him where Smithville, Texas is, he said it's about a few minutes after you pass resume speed.
So, it's not a very well known place.
But I finally found Bass.
And he is truly an outstanding aerodynamicist.
And the thing that is so important is that aerodynamics, to me, from my vantage point, is really the linchpin, you might say, of putting a system together.
Aerodynamics makes the system come alive.
And as you could hear from the various speakers, from Tom Moser, and we talked a little bit about the guidance, navigation and control, it's important for the structures.
Aerodynamics is important for the structures, it's important for the flight control system, it's important for the hydraulic system and it's important for the heating system.
So, you have to understand the aerodynamics before you can make the vehicle actually come alive.
And Bass Redd is the man to do it.
He is absolutely one of the great aerodynamicists of our times.
And he started out before there was computational fluid dynamics and the old wind tunnel testing.
And now there is computational fluid dynamics, so you're going to be in for a real treat today to hear how Bass Redd put both the Apollo together, he did the Apollo aerodynamics, and he also did the Shuttle aerodynamics.
And he is also now consulting to help NASA do their future work.
Let me now turn it over to Bass.
Thank you, Aaron.
It's a great honor to be with you this morning.
And you sure do look young.
[LAUGHTER] We will try to cover basically -- [STATIC OBSCURES] And with that overview then of the Orbiter is how the program requirements drove the aerodynamic configuration, probably the biggest thing in what the aerodynamic characteristics are driven by the program requirements.
And that will make a little more sense after we get into the briefing.
Then the aerodynamic considerations, those that aren't due to program requirements but just due to the basic fundamentals of aerodynamics and the configuration that is being evaluated.
We will do that for both the Orbiter and the Integrated Vehicle.
Then I'll try to tell you how the aerodynamic design was accomplished through each of those phases, a lot of ways to work problems.
Early on having powerful tools or sometimes a curse to you because you can produce more data than the system can consume.
And I remember when we first got started on the Shuttle design, the people from structures had just finished the NASTRAN on the Apollo and they immediately wanted enough data to put into the NASTRAN for every configuration that was being conceived.
That's not really a smart thing to do when you have literally hundreds of configurations to be considered.
We will try to talk to you about phases, phase A, B and C, and defining the problem areas.
And then defining the aerodynamic characteristics and do just a quick summary of the wind tunnel test program.
And with some comments on how CFD might have driven that were it available.
Through the `60s and `80s these design studies were accomplished in phases.
Phase A is primarily in-house civil service design.
I don't know how well you understand the NASA part, what the contractor part, what the civil service part is, but phase A is primarily in-house civil service.
Whenever the contractors began to feel that this might be a real program, they began to do their in-house studies and they began to visit and give us recommendations and ideas on their own to drive that.
None of that is paid for.
That's a gratuitous kind of a thing.
And contractors have a bit of change set aside to do those kinds of studies, but it's a freebee and really a super way to do things.
And that's still going on today.
Phase B is then you give contracts for generic studies.
Scopes out the concept feasibilities and the program requirements and does those trades, does projected cost and timelines.
By the way, before I forget it, Humboldt Mandell on costing would be excellent.
We worked quite close hand-in-hand on trying to get those requirements and cost run together along with the weight.
NASA then, after they get through this phase B, they produce a detailed statement of work that is required for the phase C and D.
And then phase C and D is the design and development.
The Air Force separated those two.
NASA always sort of put those together.
So the design, development and construction.
And the contractors do the detail work.
NASA manages and checks it.
Usually the way we operate, at least in our area, the aerodynamic area, we had a crack team that would go back in and define the problem, a potential solution to that problem and then suggest to the contractor they might look at this way or that way.
But they really put the muscle to it.
They put the final studies.
They have the design documentation.
And we try to steer that train as those things happen.
In the Shuttle now, phase A, we started in 1969, just shortly after the Lunar landing, they put together a small team.
And this is at Johnson Space Craft Center.
And that's through my knothole and the way that I look at things.
But we did end up being the lead center in the shuttle.
But we started with a small E&D group that was headed by a fellow by the name of Jim
Chamberlain. Jim Chamberlain was the one that was responsible for the Gemini Space Craft, a very unique individual gone to heaven to be with the Lord now, reviewed by Max Faget who was head of E&D and Bob Gilruth who was center director, but that was a real privilege to work with him.
What came out of that initial phase A study is what the vehicle should be, is a small personnel vehicle with a four man crew, 10k payload landing on the runway with about 200 nautical miles cross-range.
Quite different from the shuttle that we have today, but that was during the phase A.
Strong design modules in this time were developed.
And, showing how the other program requirements go with the design, the size and the program cost, there were individuals who had in their databank, which consisted of books that they kept on their tables, were all of the aircraft, all of the spacecraft, all of the missiles, what the weight of every individual subsystem was based upon plots with ECLS's on how much heat they took out, on the avionics as to how many computations they made.
Well, not to chase that rabbit too far, but basically they were down to the subsystem kind of level.
And then with the primary structure, secondary structure, how that was affected by landed weights or entry weights, the thermal protection system.
And it was amazing what a good job that kind of approach would do.
And then we had point designs that were being looked at both in-house at Johnson Space Craft Center, at Marshall and by all the contractors.
And then all that data was fed into the system.
So, that was a designed database.
Some of those have been automated now and pretty good.
And they don't give you the right answers, but the answers they give you aren't very far wrong either.
They get into the ballpark for you.
Phase B started in 1971.
Something happened.
The requirements were not dictated by NASA.
The requirements were dictated by the Air Force, at least the driving requirements.
If we had our own, a small vehicle it would have satisfied that.
The Air Force requirements were 55,000 pound payload, 60 foot long payload bay, 15 foot in diameter, it had to be returned with a 1200 nautical mile cross-range.
At least 32,000 pounds of it had to be returned.
It was classified at that time.
It is no longer classified.
You can use your imagination to figure out what kind of payload that you would want to go up into a high inclination orbit and bring back in one pass.
Where that would take you and why you'd have to get back that quickly.
But those were the requirements that were fed into phase B.
Maximum reusability did not come from the Air Force.
That came from the NASA side as that was part of technology.
NASA has two basic jobs to do.
One is the design of the vehicle, the delivering of the hardware, accomplishing the mission, but the other requirement for NASA is that it increased the technology.
Going where no man has gone before, yes, but doing things that man has not done before in a better, faster way.
And so maximum reusability was a goal that the engineers were handed.
Soft ride.
They wanted to be able to a congressman or a senator up in it.
[LAUGHTER] A neat thing.
Multi-center participation.
If you ever get Hum up here, that cost you.
Any time you break a job down and give it to more than one center or more than one contractor, it's like Humpty-Dumpty, you break him apart, you've got to put him back together again.
And it takes a lot of eyes and elbows on both sides to accomplish that.
And basically, once you break something down, you've increased your cost by 30% to 40% to put it back together again.
Now, NASA has to have, under congressional mandate, contracts in every state in the union.
Multi-center participation drives a great number of things so I felt it needed to be on the chart.
But the big thing that you need to know as engineers, sometimes you cannot set your own program requirements.
They are dictated to you for strange reasons.
And you have to play the hand that you're dealt.
Phase C and D, we had four contractors that bid on phase C and D.
The interesting thing is Rockwell was the only contractor that bid within the allocated funds.
They also had the best proposal, but that was very interesting.
What they did was use the aerodynamic database that was developed in phase A and in phase B for their design analysis.
They augmented that, they did some in-house testing, and Rockwell said we can do it for that amount of money but only if you don't change anything, NASA.
And we changed things.
They had a smaller orbiter, and we rejected that very early on.
It had no capability for weight growth.
And we showed that every program that has ever existed grew in weight by at least 5%, 10%, 15%.
And so the Orbiter was sized up just a little bit larger than the one that they had proposed with their agreement.
Aaron probably had a contract change authorization because of that.
But, anyway, we did that right at the beginning.
What we did also aerodynamically during that phase B is we developed a complete database in the nation's best facility to be used for what we call verification analysis.
And that database is very well documented, and we will talk about that a little bit more.
Program requirements.
May I ask, before you go onto program requirements, could you say something about kind of, you talked about the aerodynamic database, but nowadays we've got a lot of information about hypersonic aerodynamics?
Can you say a little bit about the state of the art?
What did people really know? Yeah. As a matter of fact, I'm going to do that when we go through it by phases specifically.
I'm kind of telling it to you like a mystery story.
[LAUGHTER] The program requirements dictated the basic orbiter configuration, payload size, crew size and returning the payload bay, the engine.
Now, if you just visualize in your mind, and it's kind of a two-chart thing, let me just jump over to that since I know how to go back now.
If you've got a payload that's got to be that long and you've got a crew size and the crew takes at least one telephone booth's worth of volume per crew takes at least one telephone booths worth of volume per crew, Even Superman needed something that big.
[LAUGHTER] You've got the volume of this.
Engines in the back.
You want to return that because engines are very, very expensive.
The avionics, of course, you want to bring those back.
They are very, very expensive.
And you've got to have it pointy end first.
You looked at that and said, boy, you didn't have much choice as far as the fuselage was concerned.
And that's right, you really don't.
Now, that's pretty well set.
Now, the wing size is required to augment the body lift.
Now, if you just had the body on there, it would have to land about 300 knots.
We didn't have wheel tires and breaks to land at that those speeds so we had to have some more lift.
And that was accommodated with a wing.
Figure out what CL max is.
They're all about the same.
You like a delta wing because it's a little more nice from a heat transfer standpoint.
Sure a whole lot nicer if you've got to go through supersonic and transonic conditions at low angles of attack.
So, you've got a delta wing that you want to have on there.
You don't have much choice on the size of that.
Airflow by the way 12% thick.
That makes it nice for the structures people.
Still does a super job aerodynamically, too.
And so that's pretty well dictated.
Then the entry angle of attack is dictated by the hypersonic L/D required for the cross-range.
And you can go through your equations, Newtonian aerodynamics sign or the exposed area, and the then L/D, the tangents.
And so you've got the hypersonic aerodynamics from that angle of attack required for the cross-range.
The wing shape to balance the configuration for the aft CG and acceptable wing leading edge heating.
In other words, we can position that wing at the right place for the center of gravity that we have which, by the way, even in phase A is known pretty well didn't change much.
The control services was the magic thing.
With the payload in and out 1.5% CG changed.
There has only been one other aerodynamic vehicle that has ever been built that has a CG of 1.5% of body length.
That was a B-36.
And this vehicle had to have the 1.5% change in the center of gravity because of payload in/ payload out.
And you can try to stack it together in many different ways.
That's just as good as you can do.
That dictated the size of the control services to accommodate that.
They are very big.
They are barn doors.
It makes it nice for controlling the vehicle.
Oh, and the vertical tail, we'll talk about that.
That's for crosswind landings.
But the way, most all the vehicles that you've seen from overseas that have been proposed cannot land in a crosswind.
I don't know what they are going to do.
They don't have enough control service to land in the crosswind.
But that dictated the vertical tail size.
Now, as you kind of go around the loop on those things, some potential items that we probably want to point out here is the body shape.
We've already basically talked about it.
Nose shape, that's pretty straight forward.
The basic Orbiter shape didn't really change from phase A through phase C and D.
We blended the OMS pot a little bit because we didn't want it to interfere with the payload bay door which was built by a different set of groups.
The delta wing, we put a little crank in it, a double delta, and got a little more subsonic lift.
And we didn't have the body flap moving to start with, but we found that it was a very powerful control of which assisted the center of gravity movement.
The full-spanned elevons were needed to control the center of pressure for hypersonics.
And there are other little things there, and you can read those through at your convenience, but those are just basic things that you have to do.
And we will comment on some of those a little bit later.
The Integrated Vehicle, the launch vehicle, if you please, what do the program requirements do to it?
Well, the Orbiter was sized for that payload bay weight.
It had to land.
It had to enter.
We knew the weights pretty well.
The 150k pound Orbiter, the 65k pound payload dictated the requirements of the Orbiter injected weight.
You add them up.
You've got to get it into orbit.
Once around at the high inclination and return to the launch site set the Orbiter performance requirements.
We had to have a certain amount of energy available from the propulsion system.
Maximum hardware reusability mixed with a cost consideration yielded a stage and a half vehicle.
And I will talk to you about that in just a minute.
But the cost constraints, there was a great move afoot to have a return to the launch site booster, manned booster, if you please, but the cost and constraints and the size of the vehicle got pretty big.
The wing vehicle loads were significantly reduced by the parallel burn.
You've got the orbiter tucked in between the solid rocket boosters and the external tank versus an orbiter that would be on the end.
It makes a great difference in the structural loads that the aerodynamics produces.
Solid rocket booster mismatch decreased or dictated an increased beta dispersion.
Typically on an end-to-end vehicle you've got everything kind of tucked in pretty tightly.
On this one the SRBs are spread, any mismatch at burnout and we had some big beta dispersions that still haunt us a little bit today.
Soft ride requirement.
Yes, I'm sorry.
I just don't know what a beta dispersion is.
You don't know what a beta dispersion is.
All right.
[LAUGHTER] Angle of attack.
Angle the size of it, beta.
Alpha, beta, standard aerodynamic definition.
Which, by the way, brings me to a comment.
We've been asked to chase a rabbit with you here.
I would suggest very highly that you get yourself a NASA dictionary where standard terms that your bosses will use because they've been using them for years.
[LAUGHTER] That you do that.
And I'm not fussing at you at all.
You can get a little gray book that's called the NASA Dictionary.
And you will know what alpha and betas are.
CMQ plus CM alpha dots.
All those standards terms are defined.
Flutter.
Buffet.
And you will really look sharp when you know what he's saying.
[LAUGHTER] All right.
Go ahead.
I know there are some people here who didn't go through aero-astro, and so may have actually not had a full course in aerodynamics.
Hopefully most of you have had it.
But any time there are expressions or terms you don't understand just do ask and we'll bring you up to speed.
And that NASA dictionary, by the way, covers not just aerodynamics.
It covers all kinds of other vocabulary kinds of things that your peers will be using and your supervisors will be using and you will know what they're talking about.
Soft ride requirements, that caused us to go to a real low dynamic pressure which is nice.
Most vehicles are up around 1200 pounds per square foot dynamic pressure.
This one is down around 800.
And the reason that came about is lesser G loads early on.
SRB flare angle, no big deal there.
Plume effects have really ate up aerodynamics.
To this day that is the only thing aerodynamically that we still don't know how to predict or the base pressure due to plume effects through the subsonic and supersonic speed regime.
CFD gets it right every now and then.
Sometimes it doesn't get it right.
And the wind tunnel test still hadn't got it right with solid plumes.
All the scaling parameters that you use still don't produce, to me, what a real valid answer is.
The flight data is used, and we update that.
Yes, sir.
When you say flare angle, do you mean like in the stack away from the Orbiter?
Yes, sir. This flare angle right back here.
We found that if you set that right, it pretty well sets the flow and the plumes behave themselves.
If you set that too small the plumes will change greatly with mach numbers, sometimes in an unpredictable fashion, so that SRB skirt size was primarily from that consideration.
This is real precious to Aaron's heart.
We changed the tank shape early in phase C and D.
And remember I told you that we had developed a database during phase B that would suffice and we could go immediately into the design and development of the total stack?
By the way, I like the ogive.
It's a lot prettier than the cone that we had on the front end.
But basically what that did, that changed the aerodynamics of the whole stack.
And the data that was delivered to structure for their structural analysis, the data that was delivered to the heating people for their heat transfer analysis had to be done all over again.
That's a year and a half cycle from the time you say let's go build a model, let's get the data, let's analyze it.
The other thing that happened when we changed the tank, everybody took note of that, and there are all kinds of other small changes that jumped on.
[LAUGHTER] On the Orbiter a little bit, on the external tank, on the SRBs and on the inclination that we had between the Orbiter and the tank.
Let me just hit some things I think that you want to take note of on this one.
I guess the nose shape, we mentioned that one already, reduces the drag.
It also reduces the loads.
We have to worry about the punch loads between the SRB and the tank.
And those aerodynamics are driven quite a bit by that nose shape.
The SRB locations fore and aft, there is some adjustment that you can have in that, and those are all optimized that whenever we do the aerodynamics that puts a minimum amount of load into the system.
Orbiter instance angle, that's the angle between the Orbiter and the external tank.
It drives the stability of the configuration to some degree.
But, more than that, it drove the loads quite a bit into the program.
And that was optimized.
Full span elevators, we use those to adjust the pitching moments so we get minimum change required to trim the vehicle from the SRBs, solid rocket boosters, and the SSMEs, the main shuttle engines.
SSME, plume effects and the plume effects from the SRB, that's the only thing that we got a bad grade on as far as predicting aerodynamics on either the Orbiter or the Integrated Vehicle.
All the rest of the stuff came out just about as we had predicted.
Now, getting back to where you asked the question.
Phase A.
Go back now.
What is a phase A?
That is the conceptual design.
We had all kinds of configurations because we had all kinds of requirements.
We really didn't know what those were, and so that analysis had to be done.
Many, many vehicles.
A lot of considerations.
The analytical techniques we used to identify problem areas.
Hypersonics, quite frankly in phase A.
Newtonian vehicle shape was approximated with flat plates.
Works great on a configuration.
[LAUGHTER] That's pretty much like a vehicle that is kind of trimmed out of a piece of a sphere, if you please.
And those aerodynamic characteristics were very good.
As a matter of fact, the thing is laid out on graph paper and you counted the squares and saw where the CG was and what things had to be.
And it worked.
It worked.
Now, we didn't know that it was working at that time.
But we found out later that it did.
But that's what we used.
[LAUGHTER] NASA documentation.
NASA had pretty good TNs on vehicles that were somewhat similar.
And the characteristics of those, we made the adjustments because of the difference in configuration.
The Air Force had a document called Dataman.
It was unpublished at that time, but we got a preliminary draft that we worked through by hand.
By the way, this is a published thing.
I would suggest very highly if aerodynamics is going to be your forte is that you learn how to use that program.
It's Dataman Aerodynamics Handbook.
Navel Ordinance had a handbook out.
They've never computerized theirs, but it's the Handbook of Supersonic Aerodynamics and good-old Horner's fluid dynamics lift and drag.
It is still around today, still use it, but those were the aerodynamic methods that we used during phase day.
And we also did small scale wind tunnel tests and those things that were the problem areas.
And the problem areas were primarily in the transition.
Yes, sir.
I can understand how you can do wind tunnel testing.
What about plume effects?
You said you didn't understand those.
How do you test that experimentally?
[UNINTELLIGIBLE PHRASE] No, that's done in a wind tunnel.
Some people said you need to match momentum on a scale vehicle.
Certain other characteristics.
What you'd like to do is be able to simulate it with a solid plume, so we did solid plume testing.
We had method of characteristics back then.
It took it about three weeks to run, to get the plume sizes, and they did a pretty good job.
But when you have the plume sizes you still after the base effects change in the pitching moment.
And so we did the solid plumes.
And we did gaseous plumes at Ames Research Center.
We did cold gas and hot gas.
But none of those met all the scaling parameters.
When you rolled all that up and you did the best you could, we still missed base pressure.
Base pressure was missed on Saturn 5.
Base pressure was missed on Mercury.
Base pressure was missed on Little Joe II and Little Joe I.
Every launch vehicle that I could find we missed base pressure on.
We missed base pressure on the Shuttle also.
Not a big effect, but it is embarrassing that you cannot get it right.
But this was done primarily more in phase C and D than in the phase A time period.
We just kind of said plumes are going to be about that much based upon data from other vehicles.
And then making sure that it was not a design driver or design stopper, if you please, that it wasn't going to be a problem that was so big that it changed things so greatly that your performance wasn't adequate.
And we had margins and everything.
Anyway, we did some small scale testing.
Phase B.
What did we do on the Shuttle on phase B?
We went into phase B with four major configurations.
Three externally identical configurations with swing wings.
It was called the Triamese.
And I think General Dynamics was the one that proposed that one.
They have all bought on another, and it's hard to find out who is who any longer.
But, anyway, that was one of the concepts.
Very, very expensive.
The swing wings, never really solved those problems.
Lockheed had a large single stage blended body, kind of an HL-10 looking thing with a flat bottom, and then with a large external tank around.
And I couldn't find pictures of all this to show you, but that was one that came in.
And then the two-stage fully reusable with both the first and the second stages being runway landers, a very favorite of Marshall.
And then, the one that we called the stage and a half where you're literally burning the first stage boosters and the second stage engines at the same time with the external tank, we referred to that as a stage and a half with parallel burn which is the current shuttle.
But those are the things that we started phase B in and did aerodynamic designs on all those.
How did we do that?
Well, we had gotten a little smarter.
A lot of the Newtonian programs had now been automated.
It was still pretty much a flat plate kind of a program, though, were you divided the vehicle up into a number of flat plates.
And then there was some contractor in-house wind tunnel test.
NASA did some limited tests on potential problem areas post phase B and pre phase C and D.
That was a gap between the time we put out an RFP, request for proposal, and the time that the contractors came back with a proposal.
We had narrowed it down that it would be a stage and a half.
That would be what we would write the RFP for.
We developed a large wind tunnel test program, developed a full aerodynamic database and heating database for that shuttle that was there at that time that was delivered to the contractors that were going to bid on that.
Integrated Vehicle.
We used the USF stability and control datcom.
Some contractor wind tunnel tests were done and limited NASA testing and analysis for potential problem areas.
And also we did a post phase B, full aerodynamic database for that vehicle.
Now, with that, what we're trying to do is eliminate configuration changes if at all possible.
And we accomplished that to some degree, but the change to the tank and other changes came back in that caused us some delay.
But it was minimized.
Phase C and D, configuration was baseline.
A big word here, make work changes only.
Nothing has changed because it looks better, works better.
You've got to change it or it will crash.
That's what we said.
That's not what we did.
We made some changes because they were nice.
Theoretical aerodynamic techniques were employed to ensure you never get to test the right Reynolds number.
Henceforth, your boundary layer is a little bit wrong.
Plume effects, they've got to be corrected.
So there was a lot of analytical work that was done during that phase C and D.
Let me say this.
That area of our technology was really improved.
A lot of dollars that went into that, Aaron's courtesy, that the program probably didn't need to fly the shuttle.
Now I tell him.
I didn't tell him back then, you're right.
[LAUGHTER] But, for the benefit of technology, we did that.
We pumped great money into CFD, great money into method of characteristics.
A lot of money into other techniques that were there.
And once more NASA goal was not only to do that mission but to help technology.
And the reason you can do CFD today was because the dollars that the shuttle program pumped into that.
Had they not pumped that in you would still be adding up flat plates.
[LAUGHTER] Aerodynamic variations and uncertainty were developed for all aerodynamic coefficients.
No other vehicle had really done that.
You sort of did the best that you could and said these are my aerodynamics.
This is my normal force versus angle of attack.
This is my side force coefficients, yawing moment coefficients versus beta.
And that said you would analyze that, give that to the flight control system, give that to the structures people and they would put their margins of safety in and look at it.
We were quite concerned on this vehicle, especially when it starts out at a 45 degree angle of attack.
And sometimes during the supersonic and transonic regime it transists down to a low angle of attack.
Flow is separated.
Some place in there it reattaches.
It cannot always make up its mind.
You've got to be able to control the vehicle, even though we weren't intentionally maneuvering at that time.
And so we developed these variations.
If you go back in the tunnel three times you get what?
Four different answers.
[LAUGHTER] And they're all very, very close.
If you change facilities you get slightly different answers.
We had some Reynolds level boundary layer kinds of problems between the wind tunnel and the full scale.
And so these uncertainties were developed for all the aerodynamic coefficients.
Then this data was delivered to the GN&C people where they make for sure that they can fly their system with the uncertainties.
The CN betas, yawing moment coefficient with angle of side slip, the control moment coefficients, the delta yawing moments and rolling moments due to elevon maneuvers, where the errors on those, that the control system wouldn't go berserk with any combination of those.
The same with structures and with flight dynamics.
Now, we're not living with those today.
We did flight tests to reduce the variations.
The astronauts put in PTI, pilot test inputs, where they would do it a certain way and we would pre-predict those characteristics.
Then we'd look at them post-flight.
We would pull the aerodynamic characteristics out with regression analysis techniques to say this is the aerodynamic coefficients that had to be there for that maneuver to look like it looked.
That, within itself, has some plus or minus stuff because you can change stuff a little bit and it still looks pretty good.
Those two then were combined and we had a set of reduced aerodynamics.
But today when you go to the aerodynamic data book, which we hope to be able to get you copies of, it will have not only the coefficients for all mach numbers, angles of attack, angle to slide slip, it will tell you what the uncertainty as a function of mach number angle of attack is for that.
And your system should be able to work with all those.
I want to mention this aerodynamic design substantiation report.
You won't be able to get a copy of that any place that I know of but we're going to try to get a copy to Jeff for you, and it should be available in your files.
But what that did, that goes back in, and for every coefficient, every angle of attack, how we arrived at that specific value and those specific variations using both wind tunnel and flight test.
A super document.
Not pretty but an excellent technical document.
And that was done during the flight test program and afterwards.
I thought we would stick up there the Wind Tunnel Testing Program.
That's primarily the backbone of the aerodynamic database of the shuttle both for the Orbiter and the Integrated Vehicle.
149 entry tests on the entry vehicle.
79 heating.
40 structural.
You can add them up.
Hours of wind tunnel test time 17,000.
Aaron asked me how that compared with the Apollo.
As best I remember, we did about 4,000 to 5,000 hours on the entry vehicle on Apollo.
However, Apollo didn't have all of those moveable surfaces.
Moveable surfaces are nice, do a lot of things for you, but you've got to test and make sure they're there.
And those were through various wind tunnels throughout the country?
Yes, sir.
These were the best facilities in the country.
Do they still exist?
Far up and away, yes, sir, they still exist.
The ones at Ames are still usable.
The ones at AEDC are still usable.
Unfortunately, those at Langley, even though they're there and they work, because of funding constraints, Langley has a hard time bringing them up and using them.
And that's unfortunate because they have some excellent facilities there.
And we surely used them.
In this Wind Tunnel Test Program, you can find out in a special wind tunnel testing program summary.
By the way, you can get this document pretty straightforwardly.
Ascent vehicle.
A few less hours, and that's because you don't move as many surfaces primarily.
Heating.
That's something to work on.
If you want a good project to work on, how do you take heating data in a wind tunnel on a small scale vehicle and make full scale predictions?
The reason that's near and dear to my heart, they ran a test not too long ago and they still cannot match those two.
A good PhD thesis.
Maybe you can figure out how to do that and how to do it right.
A lot of scaling parameters that are used, kind of like plumes.
You're not sure which scaling parameter really works, but there is slide test data, calorimeters, and there is fly test data and wind tunnel data.
It doesn't bother us that much because we've got enough conservatism in the system and uncertainties that we know we're safe to fly, but it does hurt your pride when you said hey, that heating rate should be 9 BTU's per foot squared per second and the fly test data said it's not but 2.
You might look good one way, you look bad another way, but if I was looking for something to get my plow into and I was interested in theoretical stuff, that's probably what I'd pick.
Total Wind Tunnel Test Program, Aaron paid for that out of his budget, by the way.
It was less than $100 million.
[LAUGHTER] In those year dollars.
But it was an excellent program.
Every test that was conducted was documented, analyzed, and we can make that available.
That concludes the things that I had to tell you.
And we hit that hour, just about right.
Do you have any questions that you'd like to ask?
If not, I'll give you a test.
Yes, sir.
[UNINTELLIGIBLE PHRASE] How do we affect the trim?
[UNINTELLIGIBLE PHRASE] Hypersonics, and when I say hypersonic, let me take it down to mach 5, was probably the best at prediction that we had.
A flat plate which the belly of the orbiter has a little bit of surface bending both in the pitch plane and in the yaw plane for stability.
If you put a big sphere or segment of a sphere, you could place the Orbiter on that and you'd say, hey.
And I know a sphere or segment of a sphere has stability.
Now, the uncertainties turn out not to be in normal forces or in even side forces.
Pitching moment is the one that you tend to miss the most.
And sometimes you don't know if you have a wrong pitching moment or a wrong center of gravity.
They both precipitate a delta pitching moment coefficient change.
The big barn doors, we have that into the system where we control not only a rate control system but an attitude control system.
And so it takes, with a 70 square foot, it just takes a small amount of change to correct any error that you might have in either CGR pitching moment.
Your control mechanism is with the big elevators and also the body flap a little bit, if you want to use that.
The reaction control system is a rate system.
And I didn't mention that.
And we intentionally put the reaction control system in the wake of the vehicle, even though, for orbital maneuvering, we have jets that are the forward firing end.
But so much uncertainty in how that changes the aerodynamics in a surface that is not a hard surface.
And that interaction, you can change things in such a way that you amplify it or reduce it.
And so we elected not to try to grapple with that problem and use just the aft jets, but it's just for a rate control system.
End pitch, that's the distance that separated that, and yaw on both sides, and in roll.
[UNINTELLIGIBLE PHRASE] X-15 was a low angle of attack airplane type configuration from mach 25 to mach 5.
You're flying a spacecraft kind of configuration where you basically roll about the velocity vector.
You want to maintain that pitch trim and roll about the velocity vector for cross-range in either direction.
Now, when you begin transition because you've got to be down here when you're landing, you pick the best place to do that.
And the best place to do that is back in the mach below 5 and, of course, above the subsonic regime.
And we try not to have any maneuvers that are required during that time period.
Any time you're going from a totally separated flow on the back of the vehicle to one that is now attached, separated flow cannot make its mind sometimes.
Does it want to be separated?
Does it want to be attached?
Not only the whole vehicle but even on small pieces of the vehicle.
And so there are uncertainties that come in there.
But that's the area.
But you've got to be sure.
And that's where we did a lot of our PTIs, by the way, as we became more and more brave to get those coefficients.
And the wind tunnel did a pretty good job on them.
Yes.
[UNINTELLIGIBLE PHRASE] What you're doing is changing the Newtonian flow now on my flat plate 70 square foot per surface.
You mean the rear-end?
The rear-end, the big end, yeah.
Now I've got a normal force coefficient sine squared to the angle.
Then I just change the angle up and reduce the forces on that, and that gives me a nose up.
I bring it down.
That gives me a nose down.
It works beautifully.
This is the body flap we're talking about.
Body flap and elevators, yeah, both.
Body flap and aft control surfaces.
Yes, sir.
You talked about the flight test portion of the aerodynamics.
What sort of planes were you using to do that?
The flight test was the shuttle itself.
After we safely entered and everything then we designed these pilot test inputs to extract aerodynamic characteristics.
The same technique that Edwards developed.
And they did develop that, by the way, on the X-15 and on other vehicles, too.
And we used that same technique.
We sent one of our men out and he spent about almost a half a year learning all their techniques, brought that back, developed that and worked with the astronauts very, very closely.
They trained for those maneuvers.
We extracted data for those maneuvers.
We would change the aerodynamic characteristics and not tell them what they are in the simulators.
And made sure that we didn't get any difficulty and made sure that the aero people could extract that data right and tell us how we changed it.
They all got good As.
And it worked very, very well.
[UNINTELLIGIBLE PHRASE] No, sir.
We did that one.
That was for the approach and landing test.
But for the entry vehicle, no, those were supersonic.
Yes, and we did hypersonic.
We're talking about tests that were done during the flights.
The first four flights were designated orbital flight tests.
And I think the PTIs actually continued well after that.
Yes.
Actually, what this has given us, in terms of a hypersonic database for a winged vehicle, is incredible.
At first they were called pilot test inputs because the pilots were doing them automatically.
And then I remember some of the pilots were saying you've got to do it very precisely and so we really have got to be concentrating on that.
And there are so many other things we have to concentrate on.
Could we possibly computerize this?
And so I don't remember which flight they started doing that, but at that point the PTIs became known as the program test inputs rather than the pilot test inputs.
You basically put in a little pitch up, pitch down and see how it affected the roll and the yaw and all these cross-coupling things at lots of different mach numbers.
And, over the course of a bunch of different flights and a lot of different PTIs, it really built up the aerodynamic coefficients at all these different mach numbers.
You might say a little bit about how we collected hypersonic test data in wind tunnels.
I mean it's not like you can just have a fan going and produce a mach 20 airflow for 20 minutes and you can play with your little thing.
Hypersonic testing was done primarily at the Ames Research Center three and a half foot facility.
We also did some up at Calspan, also used some of the Langley facilities, but the real workhorse was this three and a half foot hypersonic tunnel.
And basically you would have the air coming through the tunnel at the required conditions.
You would put the model of the vehicle, which is usually a 1.5% to 3% model in the facility.
You have what is called a six component balance that fits into the model.
That is a strain gauge relationship.
It gives you force and moments in both directions, or forces in both directions and some difference between the gauges so you can pull the pitching moments and the yawing moments and the rolling moments out of that.
You reduce it based primarily upon dynamic pressure or you say CN is equal to the normal force divided by QS, the dynamic pressure times a reference area.
Standard aerodynamics, but once more a language kind of thing where if you're in gas dynamics and not aerodynamics sometimes you don't get back over to those coefficients.
By the way, in that same document all of those terms are defined pretty well.
But you measure those with a balance.
Then the question happens, well, I wasn't at full scale Reynolds number.
And then that's where you come up with your theoretical techniques.
And you come back in and you look at it and you see that the boundary layer and the Reynolds number corrections theoretically make a very little bit of difference.
And then you go through today the CFD programs.
You say it gets about the same answer.
You go back to the flight test data and it gets about the same answer.
Basically, what you conclude is wind tunnels do a super job hypersonically for this kind of a configuration.
Now, it did good on the Apollo, it did good on the Gemini, it did good on the Mercury and it did good on the Shuttle.
Give me that configuration and I'll tell you what the hypersonic aerodynamic characteristics are.
I can tell you in phase A, and it's not going to be too much different at the end of phase B.
And maybe you don't want to hear that because maybe you were going to do great things in the hypersonic area.
But on a blunt body, which is what we're talking about, it does a super job now.
X-15, low angles of attack flying like an airplane, a different story completely.
[UNINTELLIGIBLE] configuration.
No, I wouldn't want to use just Newtonian to predict the aerodynamic characteristics hypersonically of that vehicle.
On the other hand, I wouldn't want to fly it there either.
It would burn up.
Blunt body is good.
98% of the energy goes into the shockwave.
98% of the energy goes into your vehicle when you're flying low.
A manned entry vehicle enters the earth's atmosphere.
You're going to be flying something like a segment of a sphere or at worst a right elliptical cone.
And Newtonian works good on that one, too, by the way.
We wind tunnel tested those for different configurations.
That gets your L/D on up to a 1.75 rather than 1.5.
Yes, sir.
Why couldn't any sort of straight winged configuration make the cross-range requirement?
The cross-range requirement is primarily angle of attack oriented.
The relationship between the CG payload in and payload out is a pretty good change.
And, if you had a straight wing, your straight wing cannot be placed in such a way that it trims subsonically and trims hypersonically.
Now, it can,at higher angles of attack with less L/D, you can make it work.
But down at 45 degrees you end up with a delta wing configuration.
Does that answer your question?
And that's a good exercise, though.
But you can go through it on a couple of sheets of paper and say ah-ha.
Because hypersonically you've got that CG, you've got your CP, you know where it has got to be placed, you get down to low angles of attack, you know where your CP is for that body, you know your CP is up at the quarter card and you know you've got 4.5 CGs.
It doesn't quite work.
It takes a delta to do that.
So it was more CG related than heating?
Yes, sir.
Although, the one that we mentioned, the 200 nautical mile cross-range with a straight wing, Langley did some tests on that, even at high angle of attack.
And right in where that straight wing ties back into the fuselage had super high heating rates.
But we could put little fillets in there and overcome that heating particular thing.
But, if you're going to go real fast, delta wings look better.
[LAUGHTER] All right.
Yes, ma'am.
When you were doing all this aerodynamic analysis, did you consider a perfect surface, or did you also do tests or analysis on what if the TPS fails?
Good question.
First of all, we have an external insulator.
And Bob Ried will talk to you about that, and Tom Moser probably has too.
Which meant that our main aluminum surface, which we want to keep quite cool, is different than the one that we initially designed.
We went back in, made models that simulated those differences, made calculations that simulated those differences.
They were very small corrections that needed to be made.
Then the other question that you had was suppose you lose a piece of TPS?
Which we did.
We've lost some chunks of TPS early in the program.
And you cannot do much testing on that because it's so little bitty and it's like a greased pencil kind of a thing.
But you go back in.
The first approach is we take some maximum things.
The pressure cannot be greater than total and it cannot be less than zero.
And so let's put those deltas into that area and into a nice area around there and see if it makes any difference to the aerodynamic coefficients.
And they're quite small and so we feel quite comfortable with that.
Now, from a heating, a different answer.
But, from an aerodynamic standpoint, does it affect the aerodynamics?
We scope it in that way.
It's a pretty small area.
Yes, sir.
Where was the greatest risk on the aerodynamic design?
During the first flight, was there anything you were particularly concerned about?
Oh, yeah.
[LAUGHTER] Getting it right.
I guess my biggest pucker factor is in the transitional phase.
I'd just seen so many things go wrong transonically that transonic wind tunnels miss.
It's a dynamic situation where you're moving.
And that was, personally, my greatest concern, did we get that right?
We did everything we could.
And it turned out that we did a good job, but I worried about that more than anything else.
Launch vehicle.
Primarily did we get the structural loads right and did we put enough margin in?
We did not use uncertainties in the pressure distributions.
Couldn't really figure out how to do that.
We had to have balance loads.
And if you said my CP can be higher or lower, do you put it higher over the whole vehicle or higher here and lower there?
And then you've got unbalanced loads.
But we did measure the wing bending moments, wing shears, wing torsion and also the attached loads in those.
And so we put some uncertainties on those.
And Tom has already been here.
I don't know if he mentioned how they tried to handle those.
But at least they had some of those things.
But we never figured out how to put uncertainties and pressure distributions and still be able to get all the balance loads for NASTRAN.
Maybe one of you all are sharp enough to figure out how to do something like that.
But that was the one on the ascent, had we adequately covered the uncertainties in pressure distributions with the way that we accomplished that by the uncertainties in the bending moments, torsion and shear?
Let's take a couple minute break and then we can resume with questions.
I am going to go down to my office and get one or two things which I think will be interesting that we can also talk about.
Take a minute or two.
Instead of one configuration, you've got one configuration times 5 degrees, 10 degrees, minus 5 degrees.
These split down the middle.
The same way here, body flap.
You've got one, two, three, four, five times five.
You've got 25 different configurations versus one.
And so it means more testing, but you're using the same facility.
It just means more hours of wind tunnel time, more hours of plotting and looking.
Say a few words about how you did the systems engineering or the systems integration between the aerodynamics and the flight control system [UNINTELLIGIBLE PHRASE].
All right.
Let's take flight control system.
We, in our division, were responsible for aerodynamics and flight dynamics.
And flight dynamics wasn't very well defined.
The Avionics Division was responsible for the GN&C system control system and also flight dynamics.
So, you've got to work together.
And you're going to get in one another's bailiwick.
That's part of good systems engineering.
You're going to do what the other fellow is going to redo, and he's going to do what you're going to redo.
But our responsibility was two-fold.
One is we had to have the right aerodynamic characteristics.
But, before we had the right aerodynamic characteristics, we had to have a configuration that should be able to live with a GN&C system.
The way we accomplished that is Ken Cox, is Ken going to be here with you?
No, OK.
Ken Cox was head of the Avionics and GN&C System.
He gave us a functional GN&C system.
It didn't have all the strings in it, didn't have all of the relays and all the stuff there, but if we gave it a command to change that we knew how much it would change and how long it would take it to change.
An elevator position as a for instance.
And so that was called a functional GN&C system.
It did the right functions but it didn't do it the same method that the real GN&C system did.
We would take our aerodynamics and see that it would work.
And we would be able to make the maneuvers that were required.
And we would analyze it.
Ad we would be pretty satisfied that with those aerodynamics and with the variations that we were OK.
But that didn't say the system was OK.
That just said that we had a pretty high probability of making it work.
Then we sent that aerodynamic database.
And all this is happening not ship it, throw it over the fence, but over a period of time we sent them the aerodynamic characteristics with the variations.
And then they beat it bloody flying everything they can think of with their GN&C system.
Fortunately, that worked out pretty well.
He would pick up a few things, but not anything major.
And, by the way, this is the only vehicle I know that happened that way on the Orbiter.
We didn't have any major configuration changes that we had to make once we got well into the program.
It stayed the same.
[UNINTELLIGIBLE PHRASE] That's right, yes.
And that was true.
That whole thing, not only the GN&C system but the hydraulics is when you give it the command, when you say I want a delta theta so much or delta E of so much, that it takes it about a quarter of a second to get there.
And that's a long time on a lag time when you pile all those things end to end up.
Now, structure is much the same way.
They gave us load indicators where they said we can take so much wing shear, so much wing torsion, so much wing bending, so much punch loads into the tank from the attach point in the front, so much punch loads into the tank on the backend, SRB and we have all the gimbling.
That's where we're doing the flight performance calculations, through the wind shears and the wind gust.
And we assure ourselves that we don't violate any of the load indicators.
But that does not say the vehicle is safe.
Then GN&C flies that one through that and gives all those conditions back over to the structures people, and they analyze the hound out of it.
They look at all the cracks and crevices.
And, if you have people that don't have a cooperative spirit, it will not work.
It just doesn't work.
So, you've got to learn how to get along with your buddies over on the other side.
Don't build your fiefdom up that your wall is so big and you control your own destiny because you don't.
And we had a super group of people to work with.
And, to me, that's what really made it work.
The same thing on heating, by the way.
They would give us heating indicators and we would fly it because that was a little more straightforward where they would give us the Q dot locals to Q dot references.
And we would look through all the Q dot references.
And we'd give them all the final set of trajectories and they would analyze it in detail.
But I look at it as here is a problem and I can work it like a piece of pie and go on this problem, go all the way down to the center, then go over and eat this piece and this piece.
It doesn't work that way.
You start around the edges and you cycle in on it.
You eat all the pie, but you accomplished it around the edges first and work out your bigger problems and then get down to the smaller problems.
Bass, I think the important point, too, is that no matter what system you work on, whether you work on the Apollo or you work on the Shuttle or whether you work on the new CEV, you're going to basically have to go through the same process.
It may be simpler or harder but you're going to have to go through the same process.
Absolutely.
Yes, sir.
You said there was no major configuration changes like around the program, but is there anything that you felt you were kind of stuck with like I wish we could have changed this?
Like not having the OMS pods stick out in the back like that?
What that a problem for aerodynamics?
No.
We lost the increased drag a little bit, but not enough to hurt anything.
And aerodynamics, it didn't bother me any that my L/D went down by 0.001.
But now the fellows that were flying it, they got in there, they said I can still go around the hack and I can still do all the things I want to.
But all that was done.
And once more, systems integration, you've got to include the crew.
GN&C system, it doesn't bother them in aero, it doesn't bother them in structures, but you've still got to fly.
And Dr.Gilvarry said you don't want the pilots talking bad about your airplanes in the bars so keep your crew happy.
[LAUGHTER] But, to answer your question, no, not really.
To meet the requirements, it's going to look pretty much the same.
If I designed it today, OK, let's go back, the same requirements now.
If you give me a different set of requirements, it's not going to look the same.
Bass, some people wanted to put some canards [UNINTELLIGIBLE PHRASE].
canards, that's not a joke.
That was done, but it was a big argument.
A canard is an aerodynamic surface part of the center of gravity.
And the beneficial part, let me tell you the good parts about canards.
canards, subsonically, I can give a pitch up moment and increase my lift.
If it's on the aft of the vehicle when I give a pitch up moment, I decrease my lift.
So, canards are wonderful.
But what canards do is it fouls up the flow over all of the rest of your vehicle.
And so, back to here, I've got one configuration.
Unsteady aerodynamics.
Heating.
Fixed configuration.
I put a canard out there.
Now I've got two more configurations, three more configurations, four more, five more, six more.
And it does a lot of grief with vortexes and stuff like that.
Now, if you had to have it, you have to have it.
Concorde had to have it, but that didn't mean that Shuttle had to have it.
It operated fine without canards.
I sure wouldn't put canards on there, if we had another shot at it.
Yes, ma'am.
Would it be easier, faster or cheaper to design the Shuttle, the Orbiter [UNINTELLIGIBLE PHRASE]?
You ask good questions, young lady.
[LAUGHTER] Yes and no.
With the tools we have and the foreknowledge that we have, having been there before, absolutely yes.
And I don't want to be negative, but you don't have the cooperative spirit going on that you had then.
I don't know why either.
The young engineers are sharper, no ifs, and or buts.
You all know a whole lot more than I ever knew, but you don't get along as well.
[LAUGHTER] This is mine.
I don't need you here.
I don't want you here.
Get out of here.
I'm going to do it my way.
And that's just an observation I made.
It might be that my knothole is too restricted.
But it goes up to pretty high management, too.
Not just at the working level.
A second thing is they asked me to come in and consult with them.
And they had lost technical capability.
Didn't use any engineers much after a certain time on the Shuttle.
And then, after the last accident, they had to bring the engineers in.
And they said I've never looked at that and I'm on the payroll.
The capability was greatly lacking, and so we developed some ways to bring them to speed in about a year and a half period of time which happened.
Observation.
By the way, I was pleased that you all got to class on time.
I told management to send them to ethics school.
Now, if there is a meeting and you tell somebody you will be there at that time, you be there at that time.
If you tell somebody you will make a deliverable at that time, you make a deliverable at that time.
Be a man or woman of your word.
And I found that lacking.
I don't know that ethics school helped too much that we sent them all to.
Well, it did, too, because the second day of class there were, I think, ten of them late.
And he sent them back and wouldn't let them in the class.
[LAUGHTER] But learn to get along with folks.
They are your friends.
They're your buddies.
They are going to be there for a lifetime.
And, yeah, you're going to have some grief.
And, yeah, he will give you some headaches or she will give you some headaches.
But you're all on the same team, and work hard towards that.
I know you've got to grit your teeth and spit sometimes, but that's major.
And that didn't cost you anything.
About the wind tunnel testing, firstly, could you have reduced that now with CFD?
And, secondly, you showed there were several hundred tests.
Yes.
And several tens of thousands of hours.
Yes.
Does that mean each test ran for several hundred hours?
Some tests ran for hundreds of hours.
That was the total number of hours for all those tests, not a per hour basis.
But, yeah, there were some hundred hour tests that went on.
To answer your question, does CFD reduce the number of wind tunnel hours?
Three areas that we're working in right now.
One reason is to bring the engineers up to technical speed where they can stand up and be counted.
And you can ask them a technical question, they can give you a technical answer and say this is my analysis and this is how I got that.
To do that we recommended back to Johnson Spacecraft Center that you better get your engineers knowing how to do wind tunnel tests and you've got to get them to know how to make the calculations to run these programs.
You've got to be able to do all that.
That's your integration contractor.
They need to have that capability.
And they've done that and they really came up to speed.
In the midst of that, they've also increased their CFD capabilities.
And I'm very envious of what you can do with CFD.
However, it hasn't decreased the amount of runs that they're requesting.
It's increasing the amount of runs that they're requesting because they will do this little bitty analysis and here is the cable tray going down that, and they'll say does it go sonic under the cable tray?
Well, I don't know.
What does the wind tunnel test say?
Well, we just have a data point here and a data point here and our CFD shows that it can go sonic under there, we need to test that to make sure that's there.
[LAUGHTER] And so it could but I don't think it will.
I think the better your tools get you want to make sure they're right.
Now, one day I think yes, that's going to happen, but right now there is still a lot of question, even in the CFD-er's mind, am I really right?
And they've always been accused of they can tell you what the right answer is if you give it to them.
[LAUGHTER] And that's really not true any longer.
That was for a number of years.
But that CFD is so great.
And they used overflow, by the way, is the one that's primary to the big workhorse right now, and it does a super job.
Was there a question?
All right.
Any other question?
I would like to address the question that was asked about [UNINTELLIGIBLE PHRASE].
Let me not talk necessarily about the Shuttle, but let me talk about Apollo for a moment.
And I mentioned it before.
In Apollo, we went to the moon with a single string avionic system.
We had one IMU, one computer on the Command and Service Module.
We had one service propulsion system to get you out of lunar orbit or to get you into lunar orbit.
And I think today it would be very difficult to do that.
I think you're probably going to have to have multiple strings.
You're going to have an escape system.
I think, in that context, it may be more difficult.
I do think the tools you have today will make it easier, but I do think what we know today, just like Bass said on understanding a little bit about the aerodynamics, you want more.
I think in some respects -- My comment is that the next time we go to the moon we're going to find out how hard it really was because I do think it's going to be hard to do.
At least that's my personal feeling.
And I think a lot of people agree with me.
One of the toughest decisions we ever made in the Space Program, and Bass and I were talking about it at breakfast, is when we decided to do Apollo 8.
Apollo 8 was probably the most fantastic mission that we ever came up with.
That was the first time we left the influence of earth's gravity, we went into orbit around the moon and we got out of orbit of the moon.
Now, we didn't land on the moon but did everything we had to do for the first time.
And I think that was probably the boldest decision that NASA and the government ever made.
Again, I look back, and I'm not sure how we would make that decision today.
It was pretty tough.
And, of course, one man led that attack, George Low who was Manager of the Apollo Program Office at Johnson Space Center, then became Deputy Administrator and then became President of Rensselaer Polytechnic Institute.
But that was really a fantastic project.
I will comment on the programs that I worked on.
Aerodynamics on Dyna-Soar, which was a defunked Air Force manned spacecraft program.
I worked on Mercury, Gemini, Apollo and Blue Gemini.
And each time I would go onto the next program and learn more, I would say we sure were lucky.
[LAUGHTER] When I was telling you about these new young engineers now that are up to speed where they didn't want to say anything.
Now that they're crunching numbers, they got a lot of criticism for the way we did it.
[LAUGHTER] And rightfully so.
A lot of capability that is there.
And you're going to have more capability today than you had yesterday, more capability on the next program than you had on the last program.
You're going to find some things to do and be able to do it better than the last program was done.
That's both good news and bad news.
Bad news is it will cost you more to do it and the good news is it will be done a whole lot better.
All right.
Any other question?
I thought it might be interesting, just to make sure you really have an understanding of what the Shuttle has to do, to sort of take them through the entry.
And maybe, Bass, you'll make some comments about the aerodynamics and I'll talk a little bit about the operations.
You're in orbit a couple hundred miles above the ground, and the first thing that you do is basically put the Orbiter, after you get everything stowed and ready for entry, so you're essentially going backwards, face to the ground.
And you burn the OMS engines.
And just, in terms of redundancy, we've got two engines.
You can cross-feed the fuel that is inside the OMS pod.
You can cross-feed from one side to the other.
So, even if you lose one OMS engine, you can still do a de-orbit burn just on the other engine.
Of course, you will have to turn a little bit so that you can burn through the CG.
If you lose both OMS engines, you can cross-feed the propellant into the reaction control system aft jets and you can come back using those.
And if you're having a real bad day and you run out, well, you know, you might have a fuel leak, for instance, and so you don't have enough propellant back there.
Actually, there was a procedure where you then would flip over and burn the remainder of your forward RCS propellant to just slow yourself down as much as you could.
We never had to do that.
In fact, I don't think we ever lost an OMS engine.
But we practice all these things.
Anyway, the point is, one way or the other, you slow yourself down.
Here is the earth and here is your orbit.
This is grossly out of scale.
You realize that on this scale your orbit is actually about like that.
In any case, you do your burn just enough to lower your perigee just about two ground level.
And now you're essentially in free-fall.
I mean you're in free-fall in orbit, but now you're gradually getting closer to the earth.
And so what you do is you now flip over and you put yourself in a position where when you actually hit the top upper reaches of the atmosphere -- And about 400,000 feet is what we call entry interface.
And, actually, the flight control system changes at that point and the computer actually goes into a different mode.
So, at this point, you're now at a 40 degree angle.
And, as Bass said, that gives you your blunt surfaces approaching.
And basically the sensors feel the deceleration.
And that's really how you know where you are in the entry.
You sense the deceleration.
That tells you what the dynamic pressure is.
During the early part of the entry, all of your control is by the reaction control system because you don't have enough dynamic pressure on the aerodynamic surfaces to be effective.
As you get down further and further into the atmosphere -- Let's see.
I think the number that comes to mind is ten.
I assume that's ten pounds per square foot dynamic pressure.
Yes.
Is when the ailerons become effective.
And so you have what is called a blended control system.
The first thing that you can do is actually control your roll, and so you disable the roll jets and you enable the ailerons.
But the pitch and the yaw is still controlled by the RCS.
And the critical thing, like Bass was saying, is you've got to keep your angle of attack constant with respect to your velocity vector because your aerodynamic heating depends on that.
If you get down to too low an angle now you're going to heat the surfaces, the upper part of the Orbiter and you're going to burn up.
You're controlling your angle around the velocity vector.
And now you can move about that.
You have a lift vector.
And now the whole name of the game, because, remember, this is a glider.
And so energy control is what is absolutely essential to make sure that you're going to end up at the threshold of the runway at about -- You said we were aiming for 150 knots.
We never actually made it.
Actually, about 200 knots was the touchdown speed.
But pretty good anyway.
We got close.
At every phase of your entry, knowing your energy and controlling it is critical.
For instance, for whatever reason, if your burn didn't go right and you think you're going to be short of the runway, we notched down the angle of attack just a little bit.
I think about 38 degrees was about the lowest you could safely go.
And you keep your lift vector.
You basically would fly the entire entry with your lift vector going away from the earth, and that maximizes your range.
But you actually target the entry so that you have more energy than you need because it's a lot easier to bleed off energy than somehow to get it back, especially when you don't have any engines.
What you normally do then is you will basically do a roll maneuver.
And you'll be rolling so that your angle of attack, with respect to your velocity vector, is always 40 degrees.
But essentially you're rolling around the velocity vector so you can now point your lift over to the side.
And, for instance, if you didn't have enough propellant when you were burning, and so now you actually have too much energy, what you're going to do -- Or, for instance, if you have a lot of cross-range that you have to make up, you could spend your entire entry over on your side like this.
And, in fact, there was even a case which we would simulate if you have an under-burn, now you have too much energy and you have to get really get rid of a lot of energy, you could actually roll 180 degrees.
And that would be pretty sporty.
[LAUGHTER] Again, nobody has ever had to do that.
But we did simulate it.
And I don't know what you would make of the aerodynamics coming in, but the point is you have a basic design that you're working with and then you try to develop operational procedures to make the system work in as many contingencies as possible.
Anyway, that's the deal.
You basically are rolling around the velocity vector to control both your energy and your cross-range.
Let's see.
Then at 20 psf the elevons actually get pitch affectivity, and so you disable the pitch jets.
And now both the pitch and the roll are controlled by the elevons.
The vertical stabilizer, as you can see, is in the shadow.
And so, actually, you don't get yaw affectivity out of the vertical stabilizer until just about transonic and subsonic, when you actually do the final pitch-over to get ready for going into the heading alignment circle.
And that's why sometimes, if you've seen pictures of the Shuttle as it approaches the landing at Kennedy Space Center, you will see little plumes come out the side.
And that's the yaw jets and the RCS which are still firing, because it's still supersonic when it gets over the Space Center.
And, if you're on the ground, you can actually hear this double sonic boom which is pretty impressive.
And I guess you get one from the front and one from the back of the vehicle, so it's a boom boom and then it goes around.
And then, of course, you hit the atmosphere at mach 25.
And so you're going through this entire aerodynamic regime down through hypersonic, supersonic, transonic, subsonic.
And I remember, Max Faget gave us a little talk about how the aerodynamic coefficients, I mean you were talking about them, but they change at different mach numbers, right?
Oh, yes.
And we mentioned this before, you know, why you really need a fly-by-wire system on this vehicle, because the control laws that you're using to do a roll maneuver at mach 15 are different from doing a roll maneuver at mach 8.
These program test inputs, you can hardly see them.
I mean you really didn't feel them.
They were just a couple of degrees that you would move the stick, you know, just little things.
But that collected enough data to get a much better determination of these coefficients.
You're very sensitive to things like beta angle.
Probably about two degrees.
If you go off more than that.
Actually, when you read the detailed description of the loss of Columbia, when they had a penetration of the forward edge of the wing, that disturbed the airflow.
And so the control system was fighting a really valiant fight to keep Columbia on target.
And they could see that on the ground.
There was more aerodynamic surface activity and the RCS jets kicked in.
In the end, of course, you run out of control authority and the vehicle eventually diverged and it actually broke up aerodynamically.
It was not an explosion.
It just broke up aerodynamically.
The hot gases in the wing were disrupting the airflow and eventually the wing was slumping, and so things were just getting worse and worse.
And I don't know exactly what went first.
Pieces were falling off along the way.
The same thing if you read the analysis of the Challenger accident on a second-by-second basis, you'll see the main engines were adjusting their thrust and trying to keep it -- The system was really working hard just doing what it was supposed to do.
Essentially the flight control system was trying to keep it within the control boundaries, and eventually it ran out of muscle because of the other failures.
And once you go to a bad beta angle or angle of attack the vehicle breaks up aerodynamically.
Bass, you might say a word about the aerodynamic coefficients and which ones were the hardest to determine and how big [were the errors?].
OK.
Basically, aerodynamic coefficients are normal forces, side forces, rolling moments, yawing moments, pitching moments and those that change that like elevators, body flaps, rudders and speed breaks.
The coefficients, the biggest variations are in the low supersonic to transonic speed regimes primarily in the control variables.
The biggest ones were just before transition or any time that the elevators were in an up position where the flow couldn't quite decide whether to be attached or separated.
And so you want to avoid that.
Digging down, not very much variations in those at all, but coming in the up position pretty big variations in the rolling moments and yaw moments due to those.
And we tried to avoid those, of course, and henceforth with the CG location.
But those were the ones that probably were the toughest and we tried to stay away from.
Overall, hypersonics right on, subsonics second best, transonic the most difficult, low supersonic kind of in between there.
The coefficients, it's hard to talk about them on a percentage basis because CM is zero and the delta CM is an infinite percentage so you cannot really talk about them on a percentage basis.
But, when you look at the plots like CM versus alpha, very small variation after we got through with the flight test program.
Before we got through the flight test program, we probably cut the variations in half on things like pitching moments, yawing moments and rolling moments.
We did better than that on the pitching moments, yawing moments and rolling moments due to elevator positions when they were local angles of attack.
And those were cut quite significantly with the PTIs.
But still, even today, you don't just have one coefficient at one angle of attack, one angle of side slippage.
You evaluate that one plus the variation that's about it.
And I remember one of the big activities in the simulators before the first flight.
They would have the test flight crews, who were going to make these flights, do flying entries.
And they would actually vary the aerodynamic coefficients.
And, if you put in a big enough variation, three sigma and all of the parameters and they went in the wrong direction, you could always make it impossible to have a successful entry.
And so it was a real question where the managers, and Aaron, maybe you have something to say about that, you and Chris Kraft had to really make the decision when you knew enough and when you could fly safely.
You're going to hear Chris Kraft talk shortly.
But I remember after we landed the first mission there were a lot of people saying that the Shuttle wasn't going to work, all the tiles were going to come off.
The flight control system was a big issue because of the aerodynamics.
The flight control system, the aerodynamics wasn't going to work. I don't know if you've ever been to the Johnson Space Center and to the Control Room, but I was sitting at the consul with Chris Kraft and he got on the Net and said -- Because there was a lot of criticism.
A lot of people wrote letters that the Shuttle would crash and wasn't going to make it.
And he got on the Net and said we're infinitely smarter today.
And I guess that summarized it, we were infinitely smarter and we did design a good system.
And I think that about summed it up, that we were infinitely smarter.
Any more questions?
Yeah.
Actually, it's an operational question.
OK.
I'm just wondering if the entire crew is trained on the whole landing procedure.
No.
We have two pilots just like in any airplane.
Some of the people who flew center seat as flight engineers were trained pilots.
And I would have been quite comfortable if they had landed the Shuttle.
But how many failures do you prepare for?
We have two people onboard who are trained to land.
I always thought to myself, we also have this auto land software which nobody has ever used, but if somehow all the other six people were incapacitated and it was just me, I would push the auto land sequence.
[LAUGHTER] And all you have to do is put the gear down manually, and I know how to do that.
But landing the Shuttle is really very complicated.
And they train hundreds and hundreds of hours in the simulator.
Some day we could spend a whole session talking about the Shuttle Training Aircraft.
It is one thing to land in the simulator, but nobody has ever died in a simulator, number one.
And, number two, you're looking at a TV screen.
You're not actually looking out at the runway.
You don't feel the bumpy aerodynamics.
And so they developed a Shuttle Training Aircraft, which is the Gulfstream II very heavily modified.
It's actually a flying simulator.
Your final approach, because your L/D on this vehicle, I don't know what it is exactly, is about five maybe.
I think five at best, yeah.
You're coming in at about a 20 degree approach angle, which may not seem like a lot.
But a commercial airplane comes in at about three degrees and the problem is, you take a regular airplane, and you point it down at a 20 degree angle,and your airspeed is going to go above your redline.
So you've got to make enough drag to simulate the Shuttle.
There's computers inside this Shuttle training aircraft.
The left seat is set up to be just like the Shuttle cockpit, and when the pilot makes the maneuvers, it basically goes back into the computers and they work the flight control system of the gulf stream, to make the gulf stream fly like the Shuttle.
The only way that they can produce enough drag to make it go at a constant speed at a 20 degree angle, is you have full speed brakes your gear is down, you have thrust reverses you run your engines in reverse, after your on the runway to slow youself down.
On the gulf stream, they run their engines at 90% reverse thrust while they're flying in the air.
It's an extraodinary vehicle.
I've flown in the center seat just to sit in it and you may be flying on this angle but it looks like your flying like this [LAUGHTER] It's really extraodinary.
Just one thing to remind you,a week from today we've asked for the initial report outline plus some reference material.
What we want to get a sense of what is your plans, what's the scope of what you're going to be looking at, what are you going to deal with and I hope by this time that you've located some reference materials you're going to be using.
We'll be sort of spiralling in on this so the idea is we'll take a look at what your plans are and then we can work together if you have some questions or having difficulties this gives us some opportunities to talk about it to make sure you're on the right track so you'll be doing something that you can be proud of, that you'll actually learn something from, so that's due a week from today.
It would be useful for me to have those electronically so that I can send them to Professor Cohen for him to look at.
I am happy to accept hard copy as well but I want them electronically Any questions?
Have a good weekend.